Except during hurricanes and other severe storms, rainfall is usually greeted with appreciation. It nourishes plants and replenishes our drinking water. But sometimes we get a little too much rain, or it comes a little too fast, sending runoff directly into creeks and streams. This stormwater runoff often picks up pollutants such as sediment from bare fields or construction sites; oil, grease, and chemicals from parking lots; nutrients from misapplied fertilizers; and untreated waste from livestock, pets, wildlife, and humans. Even when most of the rainfall infiltrates and percolates through the soil to become groundwater, it may collect various forms of pollution, particularly nutrients from agricultural operations and failing septic systems. As both stormwater runoff and groundwater eventually return to the ocean they become carriers of pollution, which could harm environmentally and economically sensitive coastal waters.
That is why it is so important to consider hydrology, the movement of water-above and below ground-no matter where your land is. Drainage-the practice of removing excess rainfall-has long been an essential part of farming throughout North Carolina, but especially in the wetter, low-lying coastal regions. Now, as population growth is causing greater pressure to further develop rural land, it is essential to remember the long-distance consequences of changing water flow. This publication will help you understand hydrology and how it influences the effectiveness of management practices aimed at improving coastal water quality.
Basic Hydrology
North Carolina's climate is humid, which means that annual precipitation exceeds evapotranspiration (ET)-the combined moisture loss to the atmosphere comprised of evaporation from the soil and transpiration from plants. In eastern North Carolina, rainfall typically ranges from 44 to 60 inches per year depending on location, while evapotranspiration ranges from 32 to 40 inches per year. Excess rainfall, the difference between rainfall and evapotranspiration, therefore ranges from 12 to 20 inches annually. Typically 1 to 2 inches per year of this excess rainfall percolates through the soil to recharge deep groundwater aquifers. The remaining excess, regardless of land use-shopping center, road, lawn, crop field, pasture, forest, wetland, park, or ballfield-eventually returns to the ocean through surface and shallow groundwater flow processes.
The movement of excess rainfall from a site is referred to as drainage. Drainage may be comprised of surface runoff and/or subsurface flow and may occur naturally or be modified through dredging, channelization, or other land modifications. The rate at which excess rainfall leaves the land depends on many factors-rainfall amount and intensity, topography, infiltration, vegetative cover, and the distance to the drainage outlet, just to name a few. Surface runoff is more likely during intense storms or prolonged rainy periods, and during such events, streams carry large volumes of water referred to as "storm flow." In milder storms, water usually infiltrates and percolates through the soil. Much of the excess rainfall that infiltrates the soil moves laterally as shallow groundwater flow until it eventually discharges into surface waters, such as drainage ditches, streams, rivers, and ultimately the ocean. Streams' "base flow" are derived from this shallow groundwater flow component. Collectively, the process of rainfall, surface runoff, infiltration, percolation, evaporation, shallow groundwater flow, and deep groundwater recharge is referred to as the hydrologic cycle. Hydrology deals with the science of water as it relates to water occurrence, distribution, and movement. Figure 1 illustrates the hydrologic cycle for eastern North Carolina.
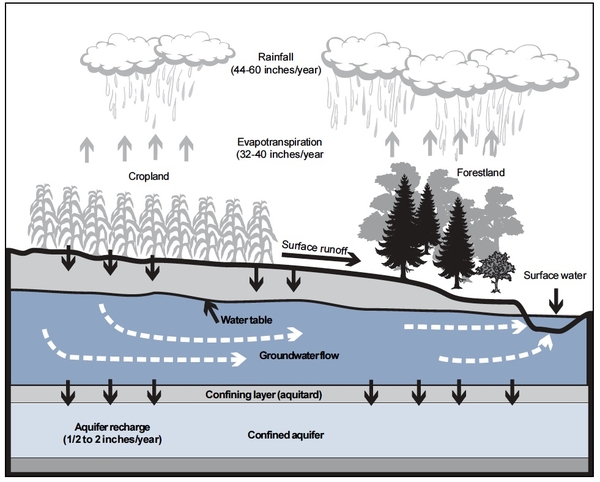
Figure 1. Basic hydrology in eastern North Carolina. Average annual rainfall ranges from 44 to 60 inches, evapotranspiration (ET) is 32 to 40 inches, deep groundwater recharge is 1/2 to 2 inches, and drainage outflow is 12 to 20 inches. Drainage outflow ultimately returns to the ocean through the surface water system, incl. drainage ditches, streams, rivers
Landscape Position, Drainage, and Soils
The cumulative natural influence of hydrology and landscape position produces two broad types of land: well-drained "uplands" and poorly drained "lowlands."
Naturally well-drained soils occupy a significant portion of the upper coastal plain and piedmont. These soils are typically found on hills or side slopes and are referred to as "uplands." The natural slope of the land results in topographic relief, which leads to rapid runoff of excess water before significant ponding can occur on the soil surface. Many well-drained soils also have good internal drainage or percolation (percolation is the ability of water to move within the soil), so rainfall infiltrates the soil quickly. The water moves vertically in deep soils or laterally in shallow soils following the natural slope of the land.
As internal drainage occurs, the emptied pores fill with air, leading to a good supply of oxygen, a condition referred to as aerobic. Aerobic conditions influence soil development and some visual soil characteristics such as color. A well-drained, aerobic environment results in high microbial activity, which leads to low levels of organic matter and thus light brown topsoil color. Bright orange, red, and yellow colors typically dominate subsoil with good drainage. Natural drainage processes on uplands remove excess rainfall in a timely manner, so soil wetness is not a significant limitation to most uses of these lands. Common uses often include agriculture, forestry, urban development, and rural building sites with good, below-ground, aerobic treatment of domestic waste.
In contrast, lower coastal plain and tidewater landscapes have significant amounts of poorly drained soils. Topography is relatively flat here, which limits surface removal of water. Furthermore, much of the region is just a few feet above sea level, so natural internal drainage is very slow. Because wetness predominates, soils often lack oxygen, which leads to an anaerobic environment. Frequent and prolonged anaerobic conditions limit oxygen-dependent microbial activity, which favors accumulation of organic matter and often leads to dark brown to black topsoil and dull orange or yellow or gray subsoil. Dull, dark soil color is a good indicator of poor drainage and prolonged wetness.
In the upper coastal plain and piedmont, poorly drained soils occupy low-lying areas and are often referred to as "lowlands." To refer to all wet soils as lowlands is sometimes misleading because a significant portion of the wettest soils in the tide-water region actually occupy the highest elevation on the landscape. This group of wet soils derives its name from an Indian term, "pocosin," meaning "swamp on a hill." They occur on broad flats often several miles from a natural stream or drainage outlet. This position is referred to as the interstream divide. Even though the interstream divide occupies the highest position in the landscape, the absolute elevation is less than 20 feet above mean sea level in the tidewater region. These soils are wet because they occur long distances from the natural stream outlets and the hills are relatively flat, resulting in very little slope—the driving force causing water to flow "down hill."
Although soils differ greatly in wetness depending on landscape position, the total volume of drainage outflow from a point on the landscape is not much different for naturally well-drained sites than for poorly drained areas. The main difference is the drainage rate and the path by which the excess water leaves the site. On well-drained sites, outflow occurs soon after rainfall and the flow doesn't take long, usually a few days. On poorly drained sites, the outflow is more gradual and usually lasts several weeks. Slow drainage often results in a shallow water table, which poses numerous land-use limitations.
Most land uses require that excess water be removed from wet soils at a faster rate than occurs naturally. On flat, wet soils, artificial drainage is therefore necessary to facilitate most land-based activities, including transportation, rural and urban development, and agriculture. The relative proportion of the North Carolina landscape requiring drainage increases as one travels from west to east. West of Interstate 95 (piedmont and upper coastal plain), only about 10 percent of the landscape is poorly drained. The middle coastal plain has about 50 percent poorly drained soils, the lower coastal plain has about 75 percent, and the tidewater region east of U.S. 17 has more than 90 percent.
Drainage and Historical Land Use
Long ago, mankind devised ways of managing water and altering hydrology by regulating its supply or removal. Egyptians and Greeks drained land as early as 400 B.C. In the coastal plain and tidewater regions of North Carolina, drainage activities predate the Revolutionary War. Many of the early settlers came from Europe where wet soils had been drained for centuries; thus, they brought a knowledge that drainage may be necessary and the methods to accomplish it. Historical records indicate George Washington and five other investors formed a company to drain 40,000 acres in the Great Dismal Swamp in 1763.
Drainage of wet soils has been a part of U.S. land policy debates since colonial times. With increasing social preference to protect wetlands, the debate has intensified in recent years. While not all "drained lands" were jurisdictional wetlands (i.e., lands protected by Section 404 of the 1972 Federal Clean Water Act), undoubtedly extensive wetlands in North Carolina were drained and converted to a variety of land uses over the past 300 years.
In the past two decades, drainage has become socially unpopular because of the perception that the only land needing drainage improvement was wetlands. In fact, many wet areas do not meet the legal definition of wetlands but require drainage improvements for effective use. The flooding of many "upland" homes, businesses, and farmlands following tropical storms and hurricanes from 1995 to 1999 raised awareness of the importance of drainage infrastructure to manage excess rainfall from all landscapes.
Nearly half of all cropland currently in production in North Carolina relies on past drainage improvement. Much of the existing drainage infrastructure-main canals and waterways-was not originally constructed to satisfy agricultural drainage requirements. Instead, early canals were dug for inland boat transportation. The canal along U.S. 17 to connect the port at Norfolk, Va., with the Albemarle Sound was proposed as early as 1784 and completed in 1812. To take advantage of the most fundamental law of hydrology, "water flows downhill," roads were constructed on piles of soil dredged from canals (spoil piles), which helped to elevate the road above the shallow water table.
Many of the roads still in use today on the Albemarle-Pamlico Peninsula were constructed on the spoil pile between two parallel canals dug primarily to provide the soil to elevate the road bed.
Eastern North Carolina still had substantial acreage of virgin timber (cypress, juniper [Atlantic white cedar], and second-growth loblolly pine) in the early 1800s. Much of it grew on the wetter soils and swampland owned by the state at the time.
The Civil War resulted in bankruptcy for many industries in North Carolina, so the state sold much of its holdings of swampland to raise money. This led to the rise of large-scale timber harvesting, but additional drainage was necessary to accommodate mechanized harvesting. Once the timber was harvested, the fertile wet soils had much potential for agriculture; but even more intensive drainage was needed for agricultural production.
U.S. policy following World War I through 1970 gave landowners incentives to complete the drainage improvements in order to provide general flood protection throughout coastal river basins and make agricultural production as efficient as possible.
The network of drainage ditches and canals on the North Carolina landscape today evolved over roughly 300 years. The previously wet soils, now drained, have much potential and are under continued pressure for a wide variety of land uses.
Agriculturally, they have become the most productive cropland in the state and account for more than 50 percent of the state's annual harvested crop yield.
Stream Channelization, Hydrology, and Water Quality
Today, most natural streams in eastern North Carolina, especially within the middle and lower coastal plain and tidewater, have been channelized. Channelization involved deepening, often straightening, and in some cases removing natural woody vegetation along the sides of the stream, an area referred to as the stream's riparian zone. In many cases, especially the smaller, "first- and second-order" streams higher in the watershed, channelization functionally disassociated the natural riparian floodplain from the stream, as shown in Figure 2. First-order streams—which are the smallest—merge into second-order streams, which flow into third-order streams, and so on.
For the floodplain to moderate and store storm flows, the stream must overflow its banks so that the water can then spread over the floor of the floodplain. In many channelized streams in the middle and lower coastal plain, most storm flows are now confined predominately to the main channel. The riparian floodplain that once routinely remained soaked or inundated during the winter and spring for months at a time now only floods during extreme storms such as hurricanes or tropical storms and remains flooded for shorter periods of time. In bypassing the floodplain, there is less opportunity for potential pollutants in the drainage water to be filtered and assimilated.
In some cases, stream channelization and removal of natural riparian vegetation have also altered water flow before it enters the stream. Drainage water from developed areas such as agricultural fields or lawns often contains nitrogen (N) and phosphorus (P). These nutrients are essential to plant growth; but excess nutrients in receiving waters can stimulate algae growth. Algae in surface water tends to deplete the oxygen necessary for fish and disrupts the aquatic food chain.
Plants cannot use all nutrients in the soil regardless of the amount or whether they occur naturally or from fertilizer application. As water percolates through the plant root zone, some nutrients dissolve and move with drainage water, eventually escaping the root zone. This process is called leaching. Although occurring in all soils, the magnitude of leaching varies dramatically depending on the nutrient; its type (nitrate-nitrogen [NO3-N], ammonium nitrogen [NH4-N], organic nitrogen, etc.); the amount present; the soils chemical, physical, and biological properties; and soil-water properties such as infiltration, percolation, wetness, and drainage intensity. As seen in Figure 3, the water draining from upland areas must pass through the riparian zone beside the stream before reaching the stream and becoming part of its base flow. During this journey, chemical, physical, and biological processes in the riparian area have the opportunity to alter the quality of the water reaching the stream.
Nitrate-nitrogen, for example, may be removed from drainage water through a biochemical process known as denitrification. During complete denitrification, soil bacteria convert water-soluble NO3-N to di-nitrogen gas (N2), which eventually returns harmlessly to the atmosphere. Denitrification only occurs under anaerobic conditions. Before channelization, a riparian area is often saturated, creating frequent anaerobic conditions in the upper soil profile. Thus, conditions for denitrification are favorable for removal of much of the nitrate-nitrogen in drainage water. Stream channelization lowers the water table, so anaerobic conditions occur less frequently in the riparian zone, as shown in Figure 4. Thus the riparian zone isn't as effective at removing nutrients, although the process still can occur during the wettest times.
An energy source, organic carbon, is needed for biological denitrification. The natural source of organic carbon is soil organic matter, which originates from the decomposition of plants and animals. Growth of new roots and die-off of existing roots are a normal, continuous process for perennial vegetation. Decaying roots provide an organic energy source for denitrification; thus, vegetation is a critical component of biological denitrification in riparian areas. Removing riparian vegetation along streams reduces the presence of the organic matter and thus may make the riparian area less effective at removing nutrients before they reach the stream. Even where vegetation is not removed, deep channelization (greater than 4 to 5 feet) may lower the flow path of shallow groundwater below the existing root zone, thus reducing the effectiveness of the riparian zone.
In the tidewater region, the hydrologic impact of stream channelization is not as great. The floodplain is bypassed for some smaller storms; but, due to the flatter gradient, water tends to back up in the channel (tailwater effect) during larger storms and overflow its banks into the floodplain so that the floodplain continues to function hydraulically even after channelization. Furthermore, on the lower floodplains, the water table remains close to the soil surface, and the amount of organic matter present is sufficiently high to avoid most of the impact of channelization on denitrification experienced in the upper basin. Finally, in the lower floodplains where the water table remains within 3 to 4 feet of the soil surface, there is less opportunity for shallow groundwater flow to bypass an active denitrification zone before entering the stream.

Figure 4. Stream channelization lowers the water table, which lowers the depth to the anaerobic zone and subsequently reduces the rate and effectiveness of denitrification in the riparian area next to the stream. Denitrification effectiveness may be further reduced by removal of riparian vegetation.
Agricultural Drainage, Hydrology, and Water Quality
The drainage intensity required for agriculture is not the same in all years or all periods of the year. While wetness is still a major concern to farmers, crops suffer from drought stresses in some years, even in traditionally wet soils. Such was the case in 1997 and in 1998 when crop yields were less than 50 percent of average due to drier than normal growing seasons that followed relatively wet springs when drainage was desperately needed to get crops planted. Intensive drainage systems that are necessary for crop production during extremely wet periods sometimes remove more water than necessary during drier periods, leading to overdrainage. Past drainage practices aimed at addressing soil wetness did not always result in the most effective holistic management of water. In recent years, emphasis has shifted from conventional drainage to water table management, often referred to as controlled drainage. It provides drainage during wet periods but also includes control structures, such as shown in Figure 5, to manage the water level at the drainage outlet, making it possible to reduce overdrainage. Regardless of land use, excess rainfall leaves a field either via surface runoff or as subsurface drainage via shallow groundwater flow. In practice, it is usually difficult to differentiate between surface and subsurface drainage because the outflow in streams, drainage ditches, or canals is a combination of both. This difference is important from a water quality and a hydrologic standpoint because the characteristics of the drainage waters differ. Subsurface drainage implies the majority of outflow has drained through the soil profile and entered the stream or channel as shallow groundwater flow. Surface drainage implies that most of the flow was across the land surface.
The following are general characteristics of drainage water based on approximately 30 years of data collected primarily in eastern North Carolina. The results are based on measurements generally conducted at the edge of fields where the outflow and constituents in the water were permanently exiting the field and potentially entering the surface water system.
- Rural land development involving improved drainage results in a 5 to 10 percent increase in total annual outflow compared to undrained forest, as shown in Figure 6. Most of the outflow from an undrained forest is surface runoff. As the drainage intensity increases, the proportion of subsurface flow increases. Increased drainage intensity results in a lower average water table depth. In rural areas without impervious surfaces (agriculture, forestry, lawns, golf courses), land use has a relatively small impact on total annual outflow. Year-to-year variation is much greater than the variability caused by changes in land use or the differences in drainage intensity.
- Peak outflow rates at the field's edge are generally two to four times higher from developed sites than from undrained sites, as shown in Figure 7. The peak outflow rate refers to the volume of water leaving a field per unit of time and is often expressed as cubic feet per second. The velocity of the flowing water, sediment transport, and stage of flow generally increase as peak flow rates increase.
- Surface drainage peak outflow rates are about two times higher than subsurface drainage rates, as shown in Figure 8. Peak outflow rates increase as the percent of impervious surface in the drainage area increases.
- Shallow groundwater below fertilized fields generally contains nitrate-nitrogen, as shown in Figure 9. Nitrate-nitrogen concentrations are generally higher from naturally well-drained sites than artificially drained sites; however, many factors interact to influence nitrate concentration. Subsurface drainage systems remove shallow groundwater, so the water contains higher concentrations of nitrates than that from surface drainage systems. But drainage waters from surface drainage systems contain higher concentrations of organic nitrogen, phosphorus, and sediment than those from subsurface drainage systems.
- Riparian buffers reduce nitrate-nitrogen concentrations entering a stream from shallow groundwater flow, as shown in Figure 10.
Total nitrogen reduction is influenced by many factors, including buffer width, depth to the water table, buffer vegetation, and soil physical and chemical properties. Riparian buffers may reduce phosphorus concentrations by about 50 percent. - Controlled drainage, when properly managed, reduces surface and subsurface drainage volume by approximately 20 to 30 percent compared to uncontrolled systems. Controlled drainage generally results in a 10 to 15 percent reduction in total nitrogen and phosphorus concentrations in drainage outflow, but variation does occur, depending on rainfall, soil type, and the proportion of surface or subsurface drainage. Controlled drainage also increases crop yield by up to 5 to 10 percent in some years, which correlates to an increase in nitrogen harvested in the crop. Combining these processes-reduced outflow volume, reduced concentration, and increased nutrient uptake by the crop-can reduce the annual transport of total nitrogen at the field edge by an average of 45 percent, as shown in Figure 11. Similarly, controlled drainage can reduce the annual transport of total phosphorus by about 35 percent, as shown in Figure 12.
Drainage water and the possible pollutants in it, whether removed by surface or subsurface drainage or whether drained naturally or artificially, eventually enter the surface water system with the potential to harm the lower estuaries and ocean sounds. The impact on the receiving water depends on its status and circulation and position within a river basin. Not all nutrients in drainage water injure the environment. Furthermore, the potential impact as measured at the "field edge" is buffered as water and pollutants move downstream before entering the more sensitive reaches of the surface water system.
Most rivers in eastern North Carolina flow from northwest to southeast. As water flows from the headwaters in the northwest to the outlet of a river basin—an estuary or the ocean—peak flow rates as measured in terms of flow stage are dampened, but the duration of flow increases. For example, along the Neuse River, a flood event that lasts one to two days in Raleigh may extend to two to three days in Smithfield, three to five days in Goldsboro, and up to a week in Kinston. Flood stages resulting from smaller storms are shorter, and larger storms last longer. As water flows through the system from a field to the lower estuary, many physical, chemical, and biological processes en route react with the flowing water to assimilate and reduce the nutrient concentrations in the flow. As was shown in Figure 10, nitrate-nitrogen concentrations in shallow groundwater originating under an agricultural field may exceed 10 milligrams per liter; but concentrations typically drop to 4 to 6 milligrams per liter in first-order streams or major canals at the edge of fields, 2 to 4 milligrams per liter in second- order streams, 1 to 2 milligrams per liter in third-and higher-order streams, and less than 1 milligram per liter in the main stem of the Neuse.
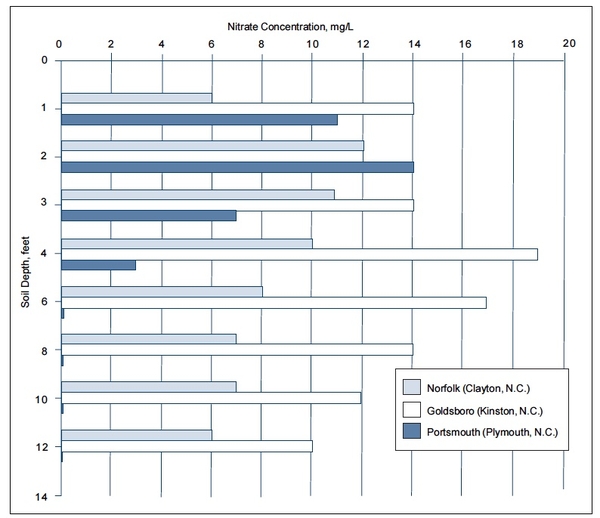
Figure 9. Typical nitrate-nitrogen concentration in shallow groundwater below agricultural fields as influenced by soil type and drainage class. Data are from a Norfolk series (well drained) near Clayton, N.C.; Goldsboro (moderately well drained) near Kinston, N.C.; and a Portsmouth (poorly drained) near Plymouth, N.C.
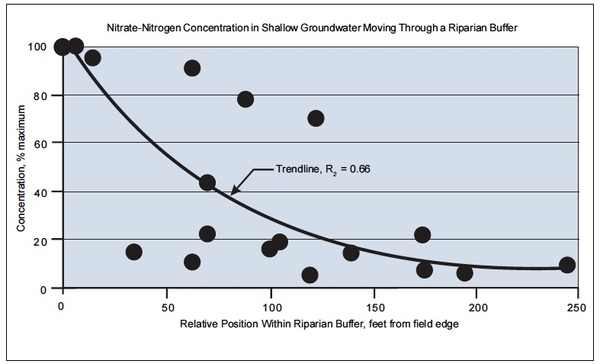
Figure 10. Nitrate-nitrogen concentration in shallow groundwater expressed as a percentage of maximum concentration (concentration at field edge). Nitrate reduction through the buffer is believed to be due primarily to denitrification occurring in the riparian zone close to the streambank.
Data taken from Jordan et al., 1993 (MD); Lowrance, 1992 (GA), Peterjohn & Correll, 1984 (MD); Jacobs & Gilliam, 1985 (NC); Buffington, 1993 (NC).
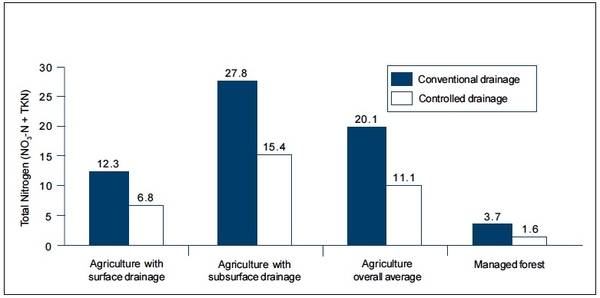
Figure 11. Average annual total nitrogen transport (TKN and No3-N) in drainage outflow as measured at the field edge of 14 sites in eastern North Carolina. Note that nitrogen transport is greater in subsurface flow than in surface runoff, and is four to five times higher in agricultural drainage than in forestland drainage.
Controlled drainage results in a net 45 percent reduction of nitrogen.
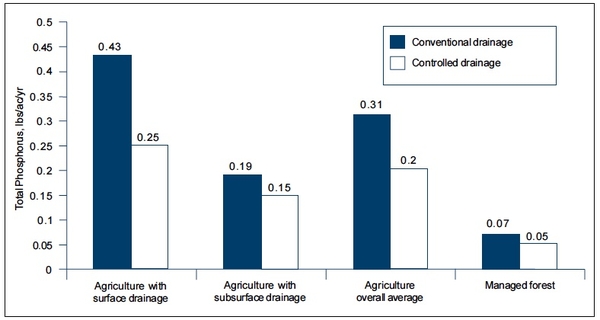
Figure 12. Average annual total phosphorus transport in drainage outflow as measured at the field edge of 12 sites in eastern North Carolina. Note that phosphorus transport is greater in surface flow than in subsurface runoff, and is four times higher in agricultural drainage than in forestland drainage.
Controlled drainage results in a net 35 percent reduction of phosphorus.
Reducing Downstream Nutrient Transport
The processes affecting nutrient transport and assimilation occur gradually. One key to helping a river system better assimilate nutrients before the nutrients reach sensitive estuaries is to slow the flow, allowing more time for assimilation to take place. Any management strategy that helps keep nutrients on-site is a positive first step. As discussed earlier, all land uses contribute flow and nutrients to the surface water system. In addition to on-site actions, off-site remedial practices may be required to deal with nutrients that escape from fields, lawns, golf courses, septic systems, parking lots, and other developed areas. A number of Best Management Practices (BMPs) can slow flow. But none of these practices is equally effective for all site and landscape conditions. In some cases, restoring the floodplain hydrology may be an appropriate action. In other cases, replanting riparian vegetation may be most effective. Under other land use and landscape conditions, controlled drainage may be the most effective and practical approach. Many situations may require a systems approach to reduce target nutrients. For BMPs to be effective, first identify and consider site-specific flow and transport processes. Only then can you select the system of BMPs with the greatest probability of reducing the transport of downstream nutrients.
If you would like more information about this subject, read the following publications:
- Agricultural Water Management for Coastal Plain Soils, AG-355
- Agriculture and Coastal Water Quality, AG-439-10
- Nitrogen Management and Water Quality, AG-439-2
- Wetland Issues, AG-439-26
2,500 copies of this public document were printed at a cost of $xxx.xx or $0.xx per copy.
Publication date: May 1, 2000
Reviewed/Revised: Sept. 26, 2024
AG-605
N.C. Cooperative Extension prohibits discrimination and harassment regardless of age, color, disability, family and marital status, gender identity, national origin, political beliefs, race, religion, sex (including pregnancy), sexual orientation and veteran status.