Silvopastures can provide multiple ecosystem services—the benefits people obtain from ecosystems—for example, forage production, meat, milk, wildlife viewing, and carbon sequestration. However, delivery of ecosystem services is mediated by specific management practices, environmental conditions, and overall design of the system. In addition to providing a general overview of silvopastures, this publication presents and discusses microclimate and forage productivity assessments (Castillo et al., 2020) of the Center for Environmental Farming Systems (CEFS) silvopasture in Goldsboro, North Carolina.
What Is a Silvopasture?
Silvopastoral systems are a type of agroforestry characterized by the intentional integration and management of trees, forages, and livestock. Silvopastures have been broadly accepted as an integrated approach to sustainable land management, while providing options to mitigate and adapt to climate change. Silvopastures have potential to deliver multiple outputs while helping to diversify farm income. For example, while grazing livestock can generate annual income, tree crops and products (for example, nuts, firewood) could also provide short- or longer-term economic output for the farm (Chizmar et al., 2020; Pizarro et al., 2020).
Silvopasture is not turning livestock loose in the woods. On the contrary, silvopastures require more intensive management, in which the various components (that is, livestock, trees, forages) and their interactions need to be accounted for and managed. For example, the arrangement of trees in the landscape is a key influential feature of silvopastures (Figure 1). Management of livestock and grazing helps to ensure uniform utilization of the forage component and to avoid areas of under- or over-use (Figure 2).
Silvopastures can be successfully established and managed by planting trees and forages at the same time, by planting trees into existing pastures, or by thinning existing tree stands and planting forages. If the goal is to keep a productive forage-livestock enterprise over the length of the rotation, lower tree densities are needed. This will require greater efforts to protect, prune, and thin the tree stand, but this intensive management has a positive trade-off in production of higher-value logs. Additional factors in thinning decisions include debris management, pruning requirements, and tree crop production.
The potential of silvopastures to deliver ecosystem services is directly related to the interaction of its components within a specific environment. Consequently, synergies and trade-offs are influenced by specific management decisions and the selection of the components. For example, in the “tall fescue” belt of the United States—the area between the temperate northeast and subtropical southeast U.S. where tall fescue is the dominant grass species for pasture-based livestock systems—the understory forage component of a system with pines as the tree component could either be a warm- or a cool-season forage (Figure 3).
What Are the Effects of Trees on Understory Forage Productivity, Microclimate, and Livestock Responses?
For many growers, trees will first be part of an animal comfort strategy—to relieve heat stress in summer or to provide windbreaks and shelter in winter—or as secondary feed sources (that is, leguminous trees). Others may be as interested in tree products, whether marketed as commodities (for example, pine straw or greenery, nuts or fruits, firewood) or as value-added goods (for example, wreaths, ciders, acorn-fattened or apple-fattened hogs). Because no tree species will meet all these criteria, selection should be a “best match” with the producer’s goals and resources.
The presence of trees in the landscape may result in competition for nutrients, water, and light, and sometimes in allelopathy (when one plant releases chemicals that hinder growth of other surrounding plants) with the companion forage species, so competition between tree growth and forage growth must be carefully managed. Physical characteristics of trees can vary. For example, some trees have deep rooting habit, late leaf out and early leaf drop, or an open narrow crown. Forages can be annual or perennial, cool or warm season. Pasture production might not be reduced when trees and forages are efficiently integrated into silvopasture systems.
In terms of livestock responses, trees in the silvopasture potentially affect microclimate, reducing heat stress for grazing livestock, specifically through shifts in understory air, temperature, humidity, and provision of shade and shelter for livestock. But the effects are variable depending on the components of the silvopasture. In addition, for a given silvopasture, the interactions among specific components can change over time, as trees grow. Although most reports on livestock responses in terms of animal weight gains or milk production have shown comparable results between treeless open pastures and silvopastures, animal welfare and reproductive performance metrics consistently improved for cattle and sheep grazing pastures with trees in warm environments (Lemes et al., 2021; Pent et al., 2020, 2021) (Figure 4).
The temperature-humidity index (THI), which combines air temperature (°F) and relative humidity (%), has been used as a measure of thermal comfort for livestock. Dairy livestock productivity may be affected when a THI threshold of 72 is reached (Igono, et al., 1992; Ravagnolo et al., 2000), resulting in economic losses (St-Pierre et al., 2003); however, the threshold varies by type of livestock (Nardone et al., 2006) and exposure time (West, 2003). In the southeastern US, THI ≥ 72 could potentially be reached for at least about a third of the year (∼100 to 150 days; Figure 5). Thorough accounting of heat stress would require weather information from the farm (as opposed to regional weather stations), thus accounting for length and strength of the heat period and information on the existence and use of heat-management measures (Ravagnolo et al., 2000). This information is of particular importance to capture the potential benefits of silvopastoral systems in the Southeast.
Forage Productivity, Light Environment, and Microclimate in a North Carolina Silvopasture
Forage accumulation, forage nutritive value, and microclimate were measured at the CEFS silvopasture in Goldsboro, North Carolina (Castillo et al., 2020). As a reference, Figure 6 provides temperature and rainfall data for the Goldsboro site. The trees were established in 2007 with an alley-cropping design (Cubbage et al., 2012). The three tree species are Pinus palustris (PP; longleaf pine), Pinus taeda (PT; loblolly pine), and Quercus pagoda (QP; cherrybark oak). The two alley-widths are 40 and 80 ft between lines of trees. Each tree-line consists of three rows of trees planted in a triangular spacing with distance between trees of 6 ft in each row, and 6 ft between tree rows (Figure 7). The forage component consists of a four-way mixture of native warm-season grasses planted in summer 2014 (Castillo et al., 2020): big bluestem (Andropogon gerardii, KY origin), eastern gamagrass (Tripsacum dactyloides, MO origin), indiangrass (Sorghastrum nutants, “NC ecotype”), and switchgrass (Panicum virgatum, Alamo). As of 2017, average tree height was 97 ft for PT, 64 ft for PP, and 50 ft for QP, and average diameter at breast height was 7.8, 5.9, and 4 inches for PT, PP, and QP, respectively.
Forage Productivity and Nutritive Value
Except for the 10-ft south sampling location (Figure 7 and Figure 8), forage accumulation was not influenced by tree species in all the sampling locations. Forage accumulation ranged from 4.3 to 5 ton/acre. Nutritive value estimates of total digestible nutrients (TDN) and crude protein (CP) were similar among sampling locations and the three tree-species systems. Concentrations of TDN and CP ranged from 54.3 to 59.3 and from 5.0 to 7.7%, respectively. According to the National Research Council (1996), the CP and TDN concentrations needed to meet the requirements of a nonpregnant nonlactating mature cow are 8.9% and 60%, respectively. The low CP and TDN concentrations in this case are due to the harvesting schedule that consisted of two clippings per year (at mid- and end-season). If the forage was grazed, grazing should be started earlier in the season, not allowing the forage to mature, and consequently resulting in greater nutritive value estimates.
Light Environment
For the CEFS silvopasture, light environment was greatest at the center of the alley (Figure 9). It was lower and varied as a function of season of the year at the 0-ft north and 0-ft south locations. The average maximum daily solar radiation per month measured at solar noon ranged from 45 to 107 watts per square foot (W/ft2), with the lower values occurring in winter (between November and February). Understory light environment [measured as relative photosynthetically active radiation (PAR) around noon] ranged from 6.3% to 99% (Figure 9). At the center of the alleys, light environment was ≥ 90% for all tree species across sampling seasons (winter and summer). In contrast, at sampling locations 0-ft north and 0-ft south, the understory light environment during summer was ≤ 15% for all tree species. During winter, however, the understory light environment was greater for QP (approximately 67% of incident PAR) compared to PP and PT (approximately 47% of incident PAR). These results highlight the potential of this specific silvopasture design (that is, alley-cropping) to provide year-round areas of high and low light (or shade) environment to protect livestock from direct and indirect solar radiation.
Temperature-Humidity Index (THI)
In general, the THI in the treeless open pasture was greater during the day (Figure 10A). Daylong (day and night) temperature, relative humidity, and THI were greater during spring and summer months than in fall and winter. Figure 10B shows the mitigation potential of trees to temperatures, relative humidity, and THI. The mitigation estimates are reported as the difference between the open pasture and under a specific tree in the corresponding time of day and year-month. There were no differences in THI mitigation among tree species, and mitigation values were greater during the summer months. The greatest mitigation values were +0.5 points during the night and -1 point during the day.
Although temperature, relative humidity, and THI had relatively moderate mitigation effects, the presence of shade (Figure 4 and Figure 9) available to grazing livestock should still be considered significant in grazing systems in the southeastern U.S. Absorption of heat from incoming solar radiation by livestock should be considered in addition to ambient air temperature. Other measures of heat stress are needed to fully characterize this phenomenon, with the ultimate effects on animal responses measured for a specific type and breed of livestock.
The effects of heat stress on livestock, and the benefits of providing shade, manifest in multiple physiological, metabolic, reproductive, and behavioral responses, as reviewed by Blackshaw and Blackshaw (1994) and West (2003). Cows provided with shade have significantly lower respiration rates and core body temperature than cattle without shade in warm summer conditions (Blackshaw and Blackshaw, 1994). Cattle’s use of shade increases as average solar radiation increases (Tucker et al., 2008), and it could reduce total heat load by 30–50% (Bond and Kelly, 1955; Bond et al., 1967). In a West Texas feedlot with artificial shade, Angus-crossbred and Charolais-crossbred heifers reached their target body weight three weeks earlier compared to unshaded cattle (Mitlöhner et al., 2020).
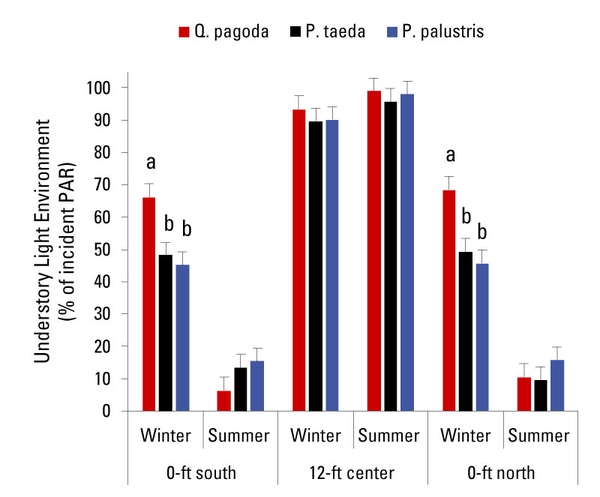
Figure 9. Understory light environment (% incident photosynthetic active radiation, PAR) as a function of tree, sampling location, and season of the year at the CEFS silvopasture in Goldsboro, North Carolina. By sampling location, bars followed by different lowercase letters are significantly different (P ≤ 0.05).
Take-Home Messages
Management is integral to the success of silvopastures. A tacit challenge for implementation of silvopastures is a willingness to manage complexity and to think longer term. Silvopastures offer great potential to diversify farm income, increase productivity, and deliver multiple ecosystem services through intentional integration and management of trees, forages, and livestock.
References
Blackshaw, J. K., and A. W. Blackshaw. 1994. “Heat Stress in Cattle and the Effect of Shade on Production and Behaviour: A Review.” Australian Journal of Experimental Agriculture 34 (2): 285. ↲
Bond, T. E., and C.F. Kelly. 1955. “The Globe Thermometer in Agriculture Research.” Agricultural Engineering 36: 251–255. ↲
Bond, T. E., C. F. Kelly, S. R. Morrison, and N. Pereira. 1967. “Solar Atmospheric and Terrestrial Radiation Received by Shaded and Unshaded Animals.” Transactions of the American Society of Agricultural Engineers 10: 622–627. ↲
Castillo, M. S., F. Tiezzi, and A. J. Franzluebbers. 2020. “Tree Species Effects on Understory Forage Productivity and Microclimate in a Silvopasture of the Southeastern USA.” Agriculture, Ecosystems & Environment 295: 106917. ↲
Chizmar, S., M. Castillo, D. Pizarro, H. Vasquez, W. Bernal, R. Rivera, E. Sills, R. Abt, R. Parajuli, and F. Cubbage. 2020. “A Discounted Cash Flow and Capital Budgeting Analysis of Silvopastoral Systems in the Amazonas Region of Peru.” Land 9 (10): Article 10. ↲
Cubbage, F., V. Glenn, J. P. Mueller, D. Robison, R. Myers, J.-M. Luginbuhl, and R. Myers. 2012. “Early Tree Growth, Crop Yields, and Estimated Returns for an Agroforestry Trial in Goldsboro, North Carolina.” Agroforestry Systems 86 (3): 323–334. ↲
Igono, M. O., G. Bjotvedt, and H. T. Sanford-Crane. 1992. “Environmental Profile and Critical Temperature Effects on Milk Production of Holstein cows in Desert Climate.” International Journal of Biometeorology 36 (2): 77–87. ↲
Lemes, Amanda Prudêncio, Alexandre Rossetto Garcia, José Ricardo Macedo Pezzopane, Felipe Zandonadi Brandão, Yeda Fumie Watanabe, Reinaldo Fernandes Cooke, Mariana Sponchiado, et al. 2021. “Silvopastoral System Is an Alternative to Improve Animal Welfare and Productive Performance in Meat Production Systems.” Scientific Reports 11 (July): 14092. ↲
National Research Council (NRC). 1996. Nutrient Requirements of Beef Cattle, 7th ed., Washington, D.C.: National Academies Press. ↲
Nardone, A., B. Ronchi, N. Lacetera, and U. Bernabucci. 2006. “Climatic Effects on Productive Traits in Livestock.” Veterinary Research Communications 30 (1): 75–81. ↲
Pent, Gabriel J., Scott P. Greiner, John F. Munsell, Benjamin F. Tracy, and John H. Fike. 2020. “Lamb Performance in Hardwood Silvopastures, II: Animal Behavior in Summer.” Translational Animal Science 4 (1): 363–75. ↲
Pent, Gabriel J., John H. Fike, and Inyoung Kim. 2021. “Ewe Lamb Vaginal Temperatures in Hardwood Silvopastures.” Agroforestry Systems 95 (1): 21–32. ↲
Pizarro, D., H. Vásquez, W. Bernal, E. A. Fuentes, J. Alegre, M. S. Castillo, and C. Gómez. 2020. “Assessment of Silvopasture Systems in the Northern Peruvian Amazon.” Agroforestry Systems 94 (1): 173–183. ↲
Ravagnolo, O., I. Misztal, and G. Hoogenboom. 2000. “Genetic Component of Heat Stress in Dairy Cattle: Development of Heat Index Function.” Journal of Dairy Science 83 (9): 2120–2125. ↲
St-Pierre, N. R., B. Cobanov, and G. Schnitkey. 2003. “Economic Losses from Heat Stress by US Livestock Industries.” Journal of Dairy Science 86, Supplement: E52–E77. ↲
Tucker, Cassandra B., Andrea R. Rogers, and Karin E. Schütz. 2008. “Effect of Solar Radiation on Dairy Cattle Behaviour, Use of Shade and Body Temperature in a Pasture-Based System.” Applied Animal Behaviour Science 109 (2): 141–54. ↲
West, J. W. 2003. “Effects of Heat-Stress on Production in Dairy Cattle.” Journal of Dairy Science 86 (6): 2131–2144. ↲
Publication date: Feb. 26, 2024
AG-959
N.C. Cooperative Extension prohibits discrimination and harassment regardless of age, color, disability, family and marital status, gender identity, national origin, political beliefs, race, religion, sex (including pregnancy), sexual orientation and veteran status.