Outline
Anatomy and Morphology—Plant Structure and Their Functions
Cells
Roots
Stems
Buds
Leaves
Flowers
Fruit
Seeds
Plant Physiology—Internal Functions and Growth
Photosynthesis
Respiration
Transpiration
Environmental Factors
Plant Growth Chemicals
Objectives
This chapter will teach people to:
- Identify and describe the function of different plant parts: leaves, buds, stems, roots, flowers, fruits, and seeds.
- Know the difference between monocots and dicots, gymnosperms and angiosperms, and annuals, perennials and biennials, as well as understand why these differences matter.
- Be familiar with plant vascular systems and why they are important.
- Understand how the environment around a plant can affect photosynthesis, respiration, and transpiration, and how the environment affects plant growth.
- Understand the effects of plant hormones on plants.
- Realize that a deeper understanding of botany helps us to identify plants, diagnose plant problems, and apply management solutions.
Introduction
Life on our planet would not be possible, much less enjoyable, without plants. They provide food either directly or indirectly to all animal life. Their leaves create oxygen, and their roots grip the soil and prevent erosion. Their branches shade and cool the ground, and they beautify our surroundings with their interesting shapes, colors, textures, and scents. Botany is the scientific study of plants. This chapter will help you understand how plants are classified, the names of their structural and reproductive components, how they grow, including the physiological processes, and how plants are influenced by environmental factors. A deep understanding of these topics will help you in diagnosing plant problems and recommending appropriate management techniques.
Botany Encompasses Several Fields
Taxonomy—Plant classification, how plants are named and grouped
Anatomy and morphology—Plant structures
Physiology—Plant internal functions and growth
Ecology—How plants interact with their environment and each other
Taxonomy—Plant Classification
More than 350,000 different types of plants live on our planet. With new species being discovered regularly and others becoming extinct, that number changes constantly (Kew Gardens). Scientists group plants that share common characteristics to make it easier to identify and study plants. This type of organization based on the characteristics of organisms is called taxonomy.
The hierarchy of classification is as follows:
- Kingdom
- Phylum
- Class
- Order
- Family
- Genus
- Species
- Variety or Cultivar
- Species
What’s in a name?
All plants have a Latin scientific name consisting of two parts, the genus and the species. This naming method is called binomial nomenclature, and it is the only consistent and dependable way to reference plants. Common names, while popular, have several shortcomings:
- Some plants have multiple common names and may be called by different common names in different states and regions. For example, the evergreen shrub Kalmia latifolia is called mountain laurel, mountain ivy, Virginia ivy, mountain kalmia, and kalmia laurel. The deciduous tree Liquidambar stryaciflua has been called American sweetgum, sweet-gum, red gum, star-leaved gum, or alligator-wood.
- The same common name is sometimes applied to several different plants. For example, tea or tea plant could be Camellia sinensis, Dysphania ambrosioides, Ilex glabra, or others.
- Some plants may have no common name.
With binomial nomenclature, it is clear you are referring to a specific plant. The first part is the generic name, which describes the genus, and the second part is the species. For the sweet gum tree Liquidambar styraciflua, the genus is Liquidamar and the species is styraciflua. A genus is a group of related species. Genera, the plural of genus, are grouped into families, families into orders, and on up the hierarchy of taxonomic classification. The scientific name is underlined or written in italics and the genus name is capitalized. In some cases, the genus may be abbreviated to the first letter followed by a period. For example, Euonymus americanus, strawberry bush, may be abbreviated as E. americanus.
Kingdom Plantae is divided into two types of land plants:
Nonvascular—Nonvascular plants are also known as bryophytes. These plants have no internal system for moving water and therefore must live in a moist environment. Because they do not have a vascular structure, they are often small. Liverworts (Figure 3–1), hornworts(Figure 3–2), and mosses (Figure 3–3) are bryophytes.
Vascular—Vascular plants contain internal vessels that can move water and nutrients throughout the plant. This system allows plants to grow quite large and to survive in many different types of ecosystems. Vascular plants are by far the most abundant type of plant on the planet. Vascular plants can be further divided into seed-producing and non-seed-producing plants. Non-seed-producing vascular plants (such as ferns and horsetails) reproduce through spores (Figure 3–4). Seed-producing vascular plants can be further divided into gymnosperms and angiosperms.
- Phylum Gymnosperm—Gymnosperm comes from the Greek word “gymnospermos,” which means “naked seed.” Gymnosperms produce seeds like angiosperms, but those seeds are not contained in a ripened ovary; they form at the tips of scales or leaves that are sometimes modified into cones. Conifers are the largest group of gymnosperms, and they bear seeds in cones. Some gymnosperms have fleshy coats or tissues surrounding seeds (yews and ginkgoes, for example).
- Phylum Angiosperm—Angiosperms are flowering plants that produce seeds contained in a fruit (a ripened ovary). Fruits have evolved to attract animals that help to disperse seeds. In addition, some fruits decompose, adding organic matter to the soil that the new plant can use to grow. Angiosperms comprise the vast majority of land plants. Flowering plants can be further divided into two classes: monocots and dicots. Some of their differences are listed in Table 3–1. We will talk more about monocots and dicots when we discuss plant anatomy and reproduction.
Table 3–1. Differences between monocots and dicots.
Class Monocots | Class Dicots |
---|---|
Embryo with single cotyledon |
Embryo with two cotyledons |
Pollen with single furrow or pore |
Pollen with three furrows or pores |
Flower parts in multiples of three |
Flower parts in multiples of four or five |
Major leaf veins parallel |
Major leaf veins netted |
Stem vascular bundles scattered |
Stem vascular bundles in a ring |
Roots that are adventitious |
Roots that develop from radicle |
Secondary growth is absent |
Secondary growth often present |
Examples: asparagus, corn, ginger, grasses, iris, lilies, onions, palms, tulips |
Examples: apples, beans, cabbage, elms, oaks, peppers, peas, potatoes, roses, spinach, squash |
The second part of the scientific name is called the specific epithet. The epithet describes the species, which is the next level of classification. Individual plants within a species have many common characteristics, yet are distinct from other species in the same genus. Specific epithets are often derived from a description of a flower or leaf, the area where the plant was discovered, or the plant's habitat; sometimes epithets also honor a person. Epithets are written in lowercase letters and are underlined or italicized (Table 3–2).
There are several small, inexpensive dictionaries on the market and websites that give the pronunciation of and a short definition for many Latin terms. Understanding the translation makes it easier to remember the scientific name. There are even sites on the Internet that spell botanical Latin names out phonetically. See the "For More Information" section at the end of this chapter for suggestions.
Two additional terms used in identifying plants are “variety” and “cultivar.” A variety is a naturally occurring subset of a species with distinctive features that are true to type, meaning that when propagated sexually, through seeds, the offspring have the same characteristics as the parent plant. The varietal name is also an epithet, added after the name of the species and preceded by the abbreviation "var." For example, Cercis canadensis var. alba is a white flowering redbud that was discovered in the wild. “Alba” means white, and seeds from this variety grow into plants that have white flowers.
A cultivar, as the name suggests, is a variety that has been cultivated by humans. Cultivars are selected for one or more unique traits and are usually propagated vegetatively to maintain these traits. If a new type of tomato were developed by crosspollination in a breeding program, it would be a cultivar. A cultivar name follows the species name and is enclosed in single quotation marks; each word begins with a capital letter. For example, Cornus florida 'White Cloud' is White Cloud flowering dogwood. It is not necessary to use the single quotes if the word "cultivar" precedes the cultivar name.
Growth Habits
Plants vary with respect to their growth and developmental cycles. Annuals complete their entire life cycle, from seed germination to seed production, and die in one growing season. Examples of annuals are corn, beans, marigolds, and zinnias. Biennials complete their life cycles in two growing seasons. In the first season, they start from seeds and produce vegetative structures and food storage organs. During the winter, a hardy evergreen rosette of leaves persists. During the second season, they flower, produce fruits and seeds, and die. Some examples of biennials are carrots, hollyhocks, celery, beets, and onions. Annuals and biennials are herbaceous plants, meaning their aboveground tissue dies back and they do not have a persistent aboveground woody stem.
Perennials live for more than two growth seasons, up to several years, decades, or even centuries. After they reach maturity, they can produce flowers and fruit each year.
Table 3–2. Examples of epithets and their translations.
Epithet | Translation |
---|---|
grandiflora |
large flower |
americana |
America |
wilsoniana |
Wilson |
autumnale |
autumn |
parvifolia |
small leaves |
japonica |
Japan |
sylvatica |
of the forest |
praecox |
very early |
Perennial plants can be either herbaceous or woody plants. Herbaceous perennials die down to the ground each winter and grow new stems from their persistent root system each spring. Several herbaceous perennials that do not tolerate cold are treated as annuals. Many bedding plants are perennial in the wild but are treated as annuals in the garden. Perennial woody plants have stems that live through the winter.
Trees are perennial woody plants that usually have one main trunk and are more than 15 feet tall at maturity. Shrubs are perennial woody plants that may have one or several main stems and are usually less than 15 feet tall at maturity.
A vine is a plant that develops long trailing stems that grow along the ground until they become supported by another plant or structure and begin to climb. Some vines have adaptive structures that help them climb: climbing hydrangea (Hydrangea anomala). develops aerial roots, and garden peas have tendrils. Vines can be annuals like sweet peas or perennials like Virginia creeper (Parthenocissus quinquefolia).
How are plants different from animals?
-
Plants use pigments in their leaves and stems to capture sunlight and create their own food through a process called photosynthesis.
-
As a product of this food production (photosynthesis), plants produce oxygen.
-
Parts of a plant, like the tips of shoots and roots (meristems), are capable of growth throughout the plant’s life.
-
Plants will respond to environmental stimuli such as animal damage or drought stress, but their response may not be evident immediately. For example, a tree that has been stressed by boring beetles might not show evidence of this infestation for several weeks or even months later.
-
Plants will grow to seal off a wound in response to injury, such as removal of a branch or bud.
Anatomy and Morphology — Plant Structure and Their Functions
Cells
Plants, like animals, consist of millions of individual cells. Both plant and animal cells contain a cell membrane, cytoplasm, a nucleus, and other organelles. Plant cells differ from animal cells in that they have a stiff cell wall and a large vacuole for storing water and other nutrients (Figure 3–5). Cell walls consist of cellulose, a type of carbohydrate that helps the plant maintain its structure. Some cells, especially those found in leaves and stems, can contain chloroplasts, which are organelles that contain cylindrical stacks called grana. In these grana is a pigment called chlorophyll that gives plants a green color and allows them to capture sunlight and turn it into sugar (energy) for the plant.
Groups of cells with similar functions are called tissues. These tissues group together to form the various parts of a plant, including roots, stems, buds, leaves, flowers, and fruits.
Roots
Roots typically develop from the lower portion of a plant or cutting. The principal functions of roots are to absorb nutrients and water, to anchor the plant in the soil or other growing medium, to provide physical support for the stem, and to serve as food storage organs. In some plants, roots are used as a means of propagation—either naturally or through human intervention.
The structure and growth habits of roots have a pronounced effect on a plant’s size and vigor, method of propagation, adaptation to certain soil types, and response to cultural practices and irrigation. Roots of certain vegetable crops are also important food sources.
Root Development
A root has three major developmental zones. The zone of cell division includes the root cap, which protects the interior cells as the root pushes through the soil, and the root apical meristem, an area of division or growth. The root apical meristem produces new cells that contribute to the root cap as well as to the root itself. The second zone, behind the meristem, is called the zone of elongation. Here cells take in food and absorb water, thereby increasing in size and pushing the tip of the root through the soil. The third major root zone is the maturation zone, where the majority of absorption occurs and cells undergo changes to become specific tissues, such as epidermis, cortex, or vascular tissue. The epidermis is the outermost layer of cells surrounding the root. These cells are responsible for absorbing water and minerals dissolved in water. The cortex is the outermost layer of the stem or root of a plant, bounded on the outside by the epidermis and on the inside by the endodermis. Vascular tissue is located in the center of the root and conducts food and water.
Why is it important to know about root hairs?
Root hairs are where the major absorption of water and fertilizer occurs. Watering and fertilizing close to the trunk of a plant are of little use to the plant, though it may help a neighboring plant. It is much more effective to apply water and fertilizer at the dripline of the plant where root hairs are most prolific.
Root hairs begin to emerge in the zone of maturation, developing as outgrowths of the epidermal cells. The majority of the water and nutrients absorbed by the plant enter through the root hairs. Most plants produce root hairs that live only a few days or a few weeks. New root hairs form as roots continue to grow through the soil. Older portions of a root do not have root hairs and cannot absorb water.
Root Systems and Adaptations
Plants generally have one of two types of root systems: taproot or fibrous root. At the end of the embryo of a seedling, the tip that will develop into a root is called a radicle. A taproot forms when the primary root continues to elongate downward into the soil. The taproot becomes the central and most important feature of the root system (Figure 3–6). Taproots have a somewhat limited amount of secondary (lateral or side) branching. Some trees, such as pecan trees, have a long taproot with very few lateral or finer, fibrous roots. This makes these trees difficult to transplant and necessitates planting in deep, welldrained soil. The taproots of carrots, parsnips, and salsify are the main edible parts of these crops.
If plants that normally develop a taproot are undercut so that the taproot is severed early in the plant's life, the root will lose its taproot characteristic and develop a fibrous root system. Undercutting is done intentionally in commercial nurseries to force trees to develop a compact, fibrous root system, which contributes to a higher rate of transplanting success.
A fibrous root system is one in which numerous lateral roots develop. These roots branch repeatedly and form the plant’s feeding root system (Figure 3–7).
Fleshy roots become food reservoirs that store surplus food for the winter or other adverse periods to be used by the plant until it is able to renew its growth. Carrots, turnips, and beets have primary or taproots containing food. Sweet potatoes and dahlia tubers are secondary roots transformed into tuberous roots packed with food.
Aerial roots form freely on some land and water plants in a favorable, moist environment (Figure 3–8). These aerial roots enable climbers such as philodendron, orchids, and air plants to attach themselves to a structure for anchorage and support. Aerial roots absorb water from the air. Many aerial roots are also adventitious, meaning they arise from stem tissue. They are often fleshy or semi-fleshy and function as reservoirs for water storage.
Factors That Influence Root Growth
- Roots grow best in soil with an adequate balance of moisture and air.
- Soil fertility and pH determine the rate and depth of root growth.
- Lack of oxygen in the soil limits growth, leading to a decrease in overall water and nutrient absorption.
- Soil compaction limits root growth.
Stems
Stems are structures that support buds, flowers, and leaves. The major internal part of a stem is its vascular tissue system, which includes xylem, phloem, and in most plants, the vascular cambium. The vascular tissue system transports food, water, and minerals and provides support for the plant. The conducting elements in xylem transport water and minerals, while those elements in phloem conduct food. Both xylem and phloem can conduct hormones.
The two classes of flowering plants, monocots and dicots, both contain xylem and phloem, but they are arranged in different patterns. In a monocot stem, the xylem and phloem are paired into discrete vascular bundles; these bundles are scattered throughout the stem like a bundle of straws (Figure 3–9).
In a dicot stem, the vascular system develops in a ring pattern (Figure 3–10). The ring of phloem is near the bark or external covering of the stem and becomes a component of bark in mature stems. In dicot woody plants, just inside the phloem is the vascular cambium, a site of cell division and active growth. The vascular cambium is responsible for a stem's increase in diameter as it produces both secondary phloem and xylem tissues. Xylem tissue forms a ring just inside the vascular cambium, thereby forming the sapwood and heartwood (Figure 3–11).
Understanding the difference between monocot and dicot vascular systems is important to gardeners for several reasons:
- A dicot tree can die if a string trimmer girdles the tree’s trunk. This can occur while someone trims the surrounding grass with a Weed Eater or other string trimmer. Such a wound can sever the vascular system just inside the bark. The same treatment of a monocot palm tree does minimal damage because the vascular system is distributed throughout the entire trunk.
- Dicots have multiple meristems, so cutting off a tip just redirects growth. Monocots, however, have only one apical meristem, so cutting the top off a palm kills the tree.
- Monocots do not have secondary meristem cells, so they grow taller but not wider. Dicots with secondary meristem cells grow wider as well as taller.
- Some selective herbicides kill only dicots.
- Other selective herbicides kill only monocots.
The stem area where leaves are attached (or were once attached) is called a node. Within the node is a bud, which is an area of great cellular activity and growth. The area between nodes is called an internode (Figure 3–12). The length of an internode depends on many factors. Low fertility, for instance, decreases internode length. Too little light causes a long internode and a spindly stem, an occurrence known as stretching or etiolation. Growth produced early in the season often results in the greatest internode length; internode length decreases as the growing season nears its end. Vigorously growing plants tend to have greater internode lengths than unhealthy plants. Internode length also varies with competition from surrounding stems or developing fruit. If the energy for a stem has to be divided between three or four stems, or if the energy is diverted into fruit growth, internode length will be shorter.
Stems can be long, with great distances between leaves and buds (such as branches of trees or runners on strawberries), or compressed, with short distances between buds or leaves (such as fruit spurs, crowns of strawberry plants, or dandelions).
Types of Stems
A shoot is a general term used to describe a young stem with leaves. A twig is a woody stem in the current or previous year's growth. A branch is a stem that is more than a year old and may have lateral stems. A trunk is the main stem of a woody plant. Woody stems contain relatively large amounts of xylem tissue in the central core and are typical of most fruit trees, ornamental trees, and shrubs.
A cane is a stem with a relatively large pith (soft spongy tissue in the center of a stem) and a life expectancy of only one or two years. Examples of plants with canes include roses, grapes, blackberries, and raspberries. Herbaceous stems contain only small amounts of xylem tissue and usually live for one growing season.
Modified Stems
When we think of a stem, we tend to picture typical trunks and branches. Modified stems can be grouped into two categories: aboveground and belowground. Aboveground modified stems are crowns, stolons, and spurs. Belowground stems are bulbs, corms, rhizomes, and tubers.
Aboveground Stems
- Crowns (on strawberries, dandelions, and African violets) are compressed stems with leaves and flowers on short internodes (Figure 3–13).
- Stolons are specialized stems that grow on the soil surface and form a daughter plantlet at one or more of their nodes. Some plants that have stolons, such as periwinkle Vinca minor, make good ground covers because they spread easily (Figure 3–14). Stolons are sometimes called runners, but technically runners are a subgroup of stolons. Examples of plants with runners are strawberries, and spider plants.
- Spurs (Figure 3–16) are short, stubby, lateral stems that arise from the main stem and are common on such fruit trees as pears, apples, and cherries. Spurs are where these trees can bear fruit. If severe pruning is done close to fruit-bearing spurs, the spurs can revert to long, non-fruiting stems.
Are palm trees made of wood? How are they different from other trees?
Along with grasses, palm trees, orchids, bamboo, irises, and yuccas belong to the class Monocotyledons (monocots) (Figure 3–15). Monocot plants grow from apical meristems and have only primary growth. Some Dicotyledons (dicots), such as oaks, maples, and elms, increase their diameter (experience secondary growth) through lateral meristems, producing wood and bark.
This secondary growth provides structural strength in dicots. How can palm trees get so tall when they do not produce wood? Their vascular bundles are tightly packed and full of cell-strengthening lignen. The bundles are surrounded by large numbers of parenchyma cells and other stem-supporting fiber. Palm trees also have leaves that are tightly packed around their stem and prop roots that help support the plant.
There are many differences between palm trees and dicot trees besides the presence of wood in dicot trees. Palms grow from a single point on the plant. If you cut the top off a palm tree, it would not produce more branches as a dicot tree would. The palm tree would die because monocots do not produce wood in concentric circles as dicots do. A monocot’s vascular bundles are scattered throughout its stem tissue. Dicot trees can be easily girdled by making a shallow cut into viable vascular bundles found just beneath the bark all the way around the trunk. Girdling can kill a dicot tree. If you made the same girdling cut around a palm tree stem, the tree would continue to live.
Woody Stem Parts and Their Functions
-
Outer bark—Protects the plant from desiccation, insects and disease, excessive heat and cold, and other injuries. Composed of cork cells that are produced from a layer of cells called the cork cambium.
- Inner bark—The interior portion of the bark, the phloem, carries food produced in the leaves down to the branches, trunk, and roots. Phloem can also move food “upward” (distal movement) to the end of a stem.
- Vascular cambium—A single layer of cells between the bark (phloem) and the wood (xylem). This layer is where growth in diameter originates. Annual rings of new wood are produced on the inside of the cambium, and new bark is produced on the outside of the cambium.
- Sapwood—The sapwood, or xylem, carries the sap (water plus nitrogen and other essential nutrients) from the roots to the leaves. Xylem also stores food synthesized in the leaves.
- Heartwood —A stem’s darker-colored center portion that was once sapwood. Heartwood is inactive wood because its cells no longer function. The core of heartwood serves only to structurally reinforce the plant and to keep it upright. Heartwood may decay over time, resulting in hollow centers in living stems.
Underground Stems
Many types of modified stems can be found beneath the soil. These belowground stems are often confused with roots. If you find the presence of buds, leaf scars, or nodes, you have an underground stem, not a root. These modified stems are energy-storage organs.
- Tubers are fleshy modified stems with nodes that produce buds (Figure 3–17). A potato is a tuber. The eyes of a potato are actually the nodes on the stem. Each eye contains a cluster of buds. A small piece of potato, as long as it contains an eye, can produce an entirely new potato plant. Some plants, such as begonias and cyclamens, produce an enlarged, modified underground tuberous stem that is short and flat. Unlike true tubers that have buds scattered on all sides, tuberous stems have shoots only on their tops.
- Rhizomes are specialized stems that grow horizontally at or just below the soil surface and serve as a storage organ and a means of propagation in some plants (Figure 3–18). Aerial stems with leaves grow from one end of the rhizome, and roots grow from the other end. Some rhizomes are compressed and fleshy, such as those of irises. They can also be slender with elongated internodes as in bent grass. Johnsongrass (Sorghum halepense) is a hated weed because of the spreading capability of its rhizomes.
- Bulbs are shortened, compressed, underground stems surrounded by thickened, fleshy leaves that envelop a central bud located at the tip of the stem (Figure 3–19). Tulips, lilies, daffodils, and onions are plants that produce bulbs. If you cut through the center of a tulip or daffodil bulb in November, you can see all the flower parts in miniature within the bulb (Figure 3–20).
- Corms are similar to bulbs in their overall shape, but when cut in half, their core is solid tissue instead layers of fleshy scales like bulbs. Corms are short, swollen stems surrounded with dead remnants of leaves or petioles that can be papery or smooth like a skin. The new stem grows from a bud on top of the corm (Figure 3–21). Some plants produce a modified stem that is referred to as a tuberous stem. Examples are crocus, tuberous begonia, and cyclamen. The stem is shortened, flattened, enlarged, and underground. Buds and shoots arise from the crown; fibrous roots occur on the bottom of the tuberous stem.
Thorns, spines, and prickles are terms you may see used interchangeably to describe the sharp projections on plants that protect them from being eaten by herbaceous animals. There are, however, botanical differences between these projections. Thorns are a modification of a stem or branch, which means they can be branched or not, have leaves or not, and they arise from a bud (Figure 3–22). A honey locust tree (Gleditsia triacanthos) has thorns. Spines are modified leaves, leaflets, petioles, or stipules (Figure 3–23). Wintergreen barberry (Berberis juliane) has spines. Despite the adage, every rose does not have thorns. The sharp projections on roses (Rosa) and blackberries (Rubus) are are modified epidural cells called prickles. Prickles can occur anywhere along the plant and are much easier to remove than thorns or spines (Figure 3–24).
Buds
A bud is an undeveloped shoot from which new leaves or flower parts arise. Buds of trees and shrubs of the temperate zone typically develop a protective outer layer of small, leathery bud scales. Annual plants and herbaceous perennials have naked buds in which the outer leaves are green and somewhat succulent. Buds are named by their location on the stem surface (Figure 3–25). Terminal buds are those located at the end of a stem. Lateral buds occur on the sides of the stem. Most lateral buds grow in the axil of a leaf (the small angle between the plant's stem and the leaf attachment) and are called axillary buds. In some instances, more than one bud is formed. Adventitious buds are those that arise at sites other than in the terminal or axillary positions. Adventitious buds may develop from the internode of the stem, at the edge of a leaf blade, from callus tissue at the cut end of a stem or root, or laterally—from a plant’s roots.
A leaf bud consists of a short stem with immature leaves (Figure 3–26). Such buds develop into leafy shoots. Leaf buds are often more pointed and less plump than flower buds.
A flower bud consists of a short stem with embryonic floral parts.
Leaves
The primary function of most leaves is to absorb sunlight for the production of plant sugars in a process called photosynthesis. Each leaf develops as a flattened surface that provides a large area for efficient absorption of light energy. The leaf is supported away from the stem by a stem-like appendage called a petiole. The base of the petiole is attached to the stem at the node.
Parts of a Leaf
The blade of a leaf is the expanded, thin structure on either side of the midrib (Figure 3–27). The blade is usually the largest and most conspicuous part of a leaf. The petiole varies in length and can be lacking entirely (described as sessile or stalkless). Stipules are leaflike structures that often occur in pairs and are sometimes fused. Stipules are usually found at the base of the petiole or on the node of a stem (as on willow, strawberry, and geranium) or occasionally fused to the petiole (as on rose, crabapple, and Callery pear). Stipules often drop as leaves mature, but those that persist or enlarge are good identification features.
The leaf blade consists of several tissue layers (Figure 3–28). The epidermis is the complex tissue layer that covers a leaf’s upper and lower surfaces and protects internal tissues. How the cells in the epidermis are arranged determines the texture of the leaf surface. Some leaves have hairs, called trichomes, that are extensions of certain cells of the epidermis. African violets have so many hairs that the leaves feel like velvet, while other species may have very few to no trichomes.
The cuticle is a thin layer covering the epidermis and consists of waxes and a substance called cutin. This substance protects the leaf from dehydration and prevents penetration of some disease-causing organisms. The amount of cuticle material produced by epidermal cells correlates to the intensity of sunlight to which leaves are exposed. Plants grown in the shade should be moved into full sunlight gradually to allow the cutin layer to build and protect leaves from the shock of rapid water loss or sunscald. The cuticle also repels water and can shed pesticides if surfactants or soaps are not used as a sticking agent. This is why many pesticide manufacturers include a spray additive that adheres to or penetrates the cuticle.
A leaf’s epidermal layer also contains small openings, each of which is called a stoma. The plural for stoma is stomata. Each stoma is surrounded by two guard cells. A stoma and its guard cells are called a stomatal complex. The bending of the two guard cells regulates the passage of water, oxygen, and carbon dioxide into or out of the leaf (Figure 3–29). The opening and closing of the stomata by their guard cells is determined by the different environmental conditions to which the leaves are exposed. Conditions that would cause a higher rate of water loss from plants (high temperature, low humidity) stimulate guard cells to close. In mild weather, stomata often remain open. With very few exceptions, guard cells usually close the stomata in the absence of light.
Leaves as a Means of Identifying Plants
Leaves, especially the shapes of their blades and margins (edges), are useful in identifying species and cultivars of horticultural plants. Simple leaves are those in which the leaf blade is a single continuous unit. A compound leaf consists of a blade that is divided into two or more similar structures called leaflets (Figure 3–30). Although compound leaves may resemble a branch stem with several leaf blades attached, leaflets do not have an axillary bud at their base. An axillary bud demarcates the true base of the entire leaf organ structure.
Most compound leaves are pinnately compound, which means that the leaf is structured like a feather, with all leaflets arranged on both sides of a central axis, called the rachis. Common examples are leaves of ashes, pecans, and Chinese pistache. Some leaves may be doubly (bipinnate) or triply (tripinnate) compound, meaning the leaflets are further divided. Honey locust and nandina (which is triply compound) are examples. Palmately compound leaves are less common than pinnately compound. Palmately compound leaves resemble a hand, with all leaflets emerging from the end of the petiole (Figure 3–31). Examples include buckeye, chaste tree, horse chestnut, and Schefflera species.
Some blades are interrupted along the margin and can be mistakenly identified as compound leaves. Lobed leaves, for example, are simple leaves with indentations reaching halfway or less to the center of the leaf, as in a pin oak. Blades with deep indentations reaching close to the midrib yet that are connected by narrow bands of blade tissue (such as ferns) are simple leaves described as pinnatifid.
Leaf Arrangement Along a Stem
Leaves are attached to stems in various patterns, and these patterns can also be used to identify plants (Figure 3–32). Alternate leaves are arranged along the stem with only one leaf at each node, alternating on each side of the stem. This arrangement is most common. Opposite leaves occur when two leaves at each node are located across from one another. A whorled arrangement is rare and requires three or more leaves at a node. Plants exhibiting whorled leaves may have opposite leaves as well, such as glossy abelia (Linnaea x grandiflora ) and cape jasmine (Gardenia jasminodies).
Venation
Plants vary with respect to the venation pattern in multiple organs, but nowhere are these differences more noticeable than in leaves. Leaves with linear venation are those with numerous lateral veins that run essentially parallel to each other and are connected laterally by minute, straight veinlets. A common type of parallel venation is that found in plants of the grass family, where the veins run parallel from the base to the apex of the leaf. Another type of parallel venation occurs in plants such as bananas, cannas, and pickerel weed. In these plants, the parallel veins run through the center then turn laterally to the margin. Leaves with parallel venation occur in monocot plants. They do not have a midrib but may have one to several larger veins with smaller parallel veins in between them.
Leaves with netted venation (Figure 3–33) have secondary veins that branch from a conspicuous midrib and then subdivide into finer veinlets, which unite into a complicated network. This system of enmeshed veins gives the leaf more resistance to tearing than most parallel-veined leaves. Netted venation may be either pinnate or palmate. In pinnate venation, the secondary veins extend laterally from the midrib to the edge, as in apple, cherry, and peach trees. Palmate venation occurs in grapevine and maple leaves, where several principal veins extend outward, like the ribs of a fan, from the petiole near the base of the leaf blade. Generally, leaves with netted venation occur on dicots.
Several distinct types of leaves occur on plants. Scale leaves or cataphylls are found on rhizomes and are also the small, leathery leaves that enclose and protect buds. Cotyledons are modified seed leaves that are part of the seed embryo and early seedling. Cotyledons typically serve as energy storage structures for germination and establishment of a seedling’s first true leaves. Other specialized leaves include sharp spines that protect the plant and tendrils that assist in supporting stems. Storage leaves are found in bulbous plants and succulents. Bracts are specialized leaves that may be brightly colored. The showy structures on dogwoods and poinsettias are not petals. They are bracts, modified to attract pollinators.
Leaf Morphology as a Means of Identification
The number of taxonomic terms can be overwhelming at first, but familiarizing yourself with them will help with plant identification. Plant identification is the first step in diagnosing any plant problem (see chapter 7, “Diagnostics”). Careful observation of plants can help us recognize the differences and similarities among plants and plant families.
Leaf Blade
These are some of the most common leaf shapes (Figure 3–34):
- Linear—Narrow, five or more times longer than wide; approximately the same width throughout
- Lanceolate—Lance-shaped; broadest below the middle and tapering toward the apex
- Elliptical—Ellipse-shaped, broadest in the middle, tapering to an acute or rounded apex and base; two or three times longer than wide
- Ovate—Egg-shaped, widest below the middle, and tapering toward the apex; two to three times longer than wide
- Cordate—Heart-shaped or broadly ovate; tapering to an acute apex, with the base turning in and forming a notch where the petiole is attached
Apexes and Bases
These are some of the most common apex (Figure 3–35) and base (Figure 3–36) shapes for leaves:
- Acuminate—A prolonged apex tapering to a long, narrow point
- Acute—Ending in an acute angle, with a sharp point
- Obtuse—Ending in an obtuse angle
- Retuse—A rounded apex with a shallow notch
- Emarginate—A deep notch
- Sagittate—Arrowhead-shaped, with two pointed lower lobes projected downward
- Truncate—Having a relatively square end
- Attenuate—A prolonged base with thin tissue extending along part of the petiole
- Cuneate—Wedge-shaped, tapering at an acute angle toward the base
Leaf Margins
Leaf margins are especially useful in the identification of some plants (Figure 3–37):
- Entire—A smooth edge with no teeth or notches
- Sinuate—Having a pronounced wavy margin
- Crenate—Having rounded teeth
- Dentate—Having large blunt teeth ending in an acute angle, pointing outward
- Serrate—Having small, sharp teeth pointing toward the apex
- Incised—Margin cut nearly to the midrib into sharp, deep, irregular incisions
- Lobed—Incisions extend less than halfway to the midrib
- Cleft—Incisions extend more than halfway to the midrib
Leaf Textures
Leaf texture is another way to identify a plant. These are some common leaf textures:
- Succulent—Fleshy, soft, and thickened in texture; modified for water storage
- Coriaceous—Leather-like, tough
- Glabrous—Smooth, shiny, hairless surface
- Downy—Covered with very short, weak, and soft hairs
- Pubescent—A hairy surface
- Tomentose—Covered with matted, wooly hairs
- Hirsute—Pubescent with coarse, stiff hairs
- Hispid —Rough with bristles, stiff hairs, or minute prickles
- Scabrous—Rough to the touch, with the texture of sandpaper
Flowers
Although we appreciate flowers for their beauty and scent, a flower’s sole function is sexual reproduction. Plants like grasses and pines that are wind pollinated have no need to attract an insect or animal and so expend little energy on beauty but instead produce copious amounts of pollen for the wind to disperse. Other plants, however, have developed fragrant, pale, night-blooming flowers to attract pollinator moths.
Most fragrant, showy flowers with nectaries have evolved to attract insect pollinators to ensure the reproductive success and continuance of the plant species. Markings on petals signal to insects how to approach and where to land. Bee-pollinated flowers reflect ultraviolet light that is visible to insects but not humans. Some male insects are tricked into transferring pollen by flowers that look and smell like females of an insect species. Fragrant flowers attract bees, butterflies, moths, and other insects. Flowers with foul odors attract flies, beetles, and similar insects.
Other animals, such as birds and bats, also pollinate flowers. Some flowers have landing platforms or nectaries at the end of long tubes to match the body parts of the animals that perform pollination.
Parts of the Flower
As the reproductive structure of the plant, the flower contains pollen-producing structures, ovule-bearing structures, and sterile structures such as petals, sepals, and nectaries (Figure 3–38).
A peduncle is a stem that supports the flower or flowers. Sepals are small, usually green, leaf-like structures on the base of the flower. Sepals collectively are called the calyx. Petals are usually the highly colored portions of the flower. They may contain specialized structures that produce molecules associated with scent called osmophores, or they may produce nectar in structures known as nectaries. The petals collectively are the corolla.
The number of petals and sepals on a flower, and the form they take, are often used to identify plant families and genera. The calyx and corolla together are called the perianth.
The receptacle is the expanded tip of a flower stalk that bears the flower’s sexual organs. The pistil, located in the center, is the female structure of a flower. Some plants have flowers with no pistil; others have one or more pistils. The totality of the pistils is referred to as a gynoecium (“house of woman”). A single pistil consists of a stigma, style, and ovary. The stigma is located at the apex and is the site of pollination. The style is the stalk between the stigma and the ovary. The ovary is the swollen part at the base of the style; it contains ovules, each of which produces a single egg cell. After the egg is fertilized, the ovule develops into a seed, and the ovary matures into the fruit.
The stamen is the male reproductive organ. Each stamen consists of an anther, containing one to several pollen-producing chambers, and a long, supporting stalk called the filament. This filament holds the anther in position so that pollen can be disbursed by wind or carried to the stigma by insects, birds, or other pollinators.
Types of Flowers
A flower that has sepals, petals, stamens, and pistils is called a complete flower. Incomplete flowers lack one or more of these parts. Grass plants, for example, typically do not have sepals or petals. If a flower contains both male and female reproductive parts, it is said to be perfect. Flowers that lack one of the reproductive structures, either the stamens or pistils, are known as imperfect flowers. An imperfect flower is also therefore, by definition, incomplete.
Some plants bear only “male” flowers (staminate) or only “female” flowers (pistillate). Species in which the sexes are separated into staminate and pistillate plants are called dioecious (two houses). Most hollies are dioecious. Therefore, to obtain holly berries, we must plant female plants with a male plant nearby. Plants that have separate male and female flowers on the same plant are monecious (one house). Corn, watermelon, and pecan trees are examples of monecious plants. Some monecious plants, such as cucumbers and squash, bear only male flowers at the beginning of the blooming season but later develop flowers of both sexes.
Types of Inflorescences
Some plants bear only one flower per stem or branch; these are called solitary flowers. Other plants produce an inflorescence, a term that refers to a cluster of flowers and how they are arranged on a floral stem (Figure 3–39). Most inflorescences can be classified into two groups, indeterminate and determinate. Indeterminate inflorescences have an axis terminating in meristematic tissue, which continues to develop until the inflorescence is complete. Flowers bloom in sequence from the bottom to the top of the axis or inward, starting from the outside like Gladiolus spp.
There are five basic types of indeterminate inflorescences. A raceme has an elongated axis with stalked flowers. A spike has an elongated axis with sessile (stalkless) flowers. A corymb has a flat-topped or hemispherical raceme with floral stalks of varying lengths arising up the axis. An umbel is similar, but the branches arise from one point at the top of the axis. A panicle is a branched raceme.
Determinate inflorescences have a flower that terminates the axis early in its development. Flowers bloom in sequence down from the tip of the axis or progressively outward from the middle. A head has all flowers attached to a disk; examples are sunflowers and Echinacea species. A cyme is a broad, flat-topped, or hemispherical inflorescence with flower clusters of threes, in which the floral stalks are similar in length. Some inflorescences can have an indeterminate central axis and determinate lateral branches, such as buckeyes.
Day Length and Flowering
Light duration, or photoperiod, refers to the amount of time that a plant is exposed to sunlight. The ability of many plants to flower is controlled by photoperiod. When scientists first recognized the concept of a photoperiod, they believed that the duration of exposure to light triggered flowering. Later they discovered that it is not the light but the uninterrupted dark that triggers floral development. Plants can be classified into three categories, depending upon their flowering response to the duration of darkness: short day, long day, or day neutral.
Short-day plants produce flowers only when the period of darkness exceeds a critical minimum called the critical night length. Short-day plants include many spring- and fall-flowering plants such as chrysanthemum and poinsettia. Long-day plants form flowers only when the period of darkness is less than the critical night length. They include almost all of the summer-flowering plants, such as black-eyed Susan (Rudbeckia fulgida) and California poppies (Eschscholzia californica), as well as many vegetables, including beets, radishes, lettuce, spinach, and potatoes.
Because chrysanthemums flower under the short-day conditions of spring or fall, the method for manipulating the plant into experiencing short days is simple. If long days are predominant, a shade cloth can be drawn over the chrysanthemum for 13 hours daily to block out light until flower buds are initiated. To bring a long-day plant into flower when sunlight is not present longer than a critical minimum, artificial light can be added until flower buds initiate.
Day-neutral plants produce flowers regardless of day length. Some plants do not really fit into any category but can respond to combinations of day lengths. Petunias will flower regardless of day length but will do so earlier and more profusely under long periods of daylight exposure (short periods of darkness).
Fruit
Fruits are important to human nutrition and often have a prominent place in a garden. A fruit is a ripened ovary that contains one or more seed. The fruit functions as protection for the seed until it is ready for dispersal. Many fruits are attractive to animals, thus a fruit’s seed can be eaten, pass through a digestive tract, and end up far away from the parent plant, spreading genes and ensuring long-term survival of the plant. Some fruits rely on wind to disperse the seeds like the fluff on dandelion or the winged samara of a maple.
Types of Fruit
Fruits can be simple or compound. Simple fruits are formed in a flower with one ovary or several fused ovaries. Cherries, nectarines, peaches, and plums are drupes with a single hard seed in the center (Figure 3–40). Apples, pears, and quince are known as pomes. Each is a single fruit, but inside it has five fused ovaries, hence the five-pointed star shape when it is cut around its equator (Figure 3–41). Cantaloupes, cucumbers, eggplant, pumpkins, tomatoes, and watermelons are considered berries; they have several seeds inside a soft outer shell (Figure 3–42). Some fruits are dry and do not have the typical flesh that comes to mind when one hears the word “fruit.” Dry fruits can be either dehiscent and open when ripe or indehiscent and remain closed when ripe. Milkweed, poppies, and legumes are examples of dehiscent fruits (Figure 3–43). Indehiscent fruits remain closed when ripe and depend on some other mechanism, such as decay or predators, to release their contents. Indehiscent fruits include pecans, peanuts, or maples (Figure 3–44).
Compound fruits are either aggregate or multiple. If a single flower has several ovaries that stay together, it forms an aggregate fruit. Raspberries are aggregate fruits, technically aggregate drupes (Figure 3–45). If several flowers are tightly clustered together on one structure and these ovaries remain close together as the fruit ripens, they produce multiple fruits. Pineapples and figs are examples of multiple fruits (Figure 3–46).
Strawberries are not BERRIES
The red part of the strawberry is, technically speaking, not a fruit; it is a ripened receptacle. If you look closely at a strawberry, you can see that each of its seeds is a mini indehiscent fruit called an achene (Figure 3–47). The receptacle ripens upward, softens, and turns red, attracting seed dispersers like birds.
Seeds
Seeds can be a flower’s end product. A seed contains three parts (Figure 3–48):
- A miniature plant (embryo) that is in a state of dormancy.
- An endosperm, which is a stored food source for the embryo that can include proteins, carbohydrates, or fats.
- A seed coat or layer around the seed that protects the embryo from diseases, insects, and moisture until it is time for germination.
Germination occurs when the proper temperature, moisture, oxygen, and light conditions are met. As water is drawn in through the seed coat, the radicle is the first part of the seed to appear. The radicle will develop into the primary root and will eventually sprout root hairs and lateral roots. The hypocotyl will eventually become the stem. The first leaves or cotyledons are usually shaped differently than the leaves the mature plant will produce. Those seeds with two cotyledons are dicots and those with one are monocots.
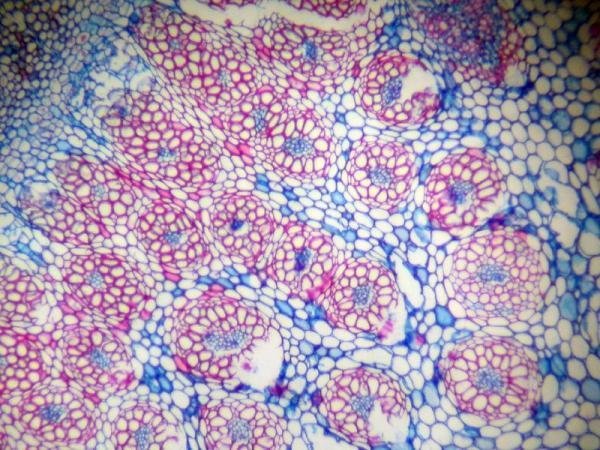
Figure 3–9. Monocot stem cross section showing vascular bundles randomly scattered.
Andrea Scauri, Flickr CC BY 2.0
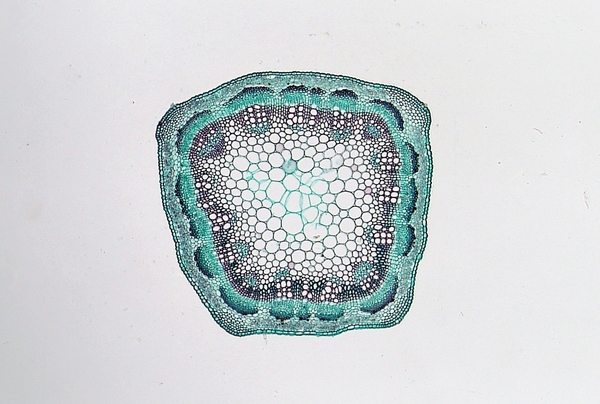
Figure 3–10. Dicot stem cross section showing vascular bundles in a ring.
Berkshire Community College, Flickr CC0
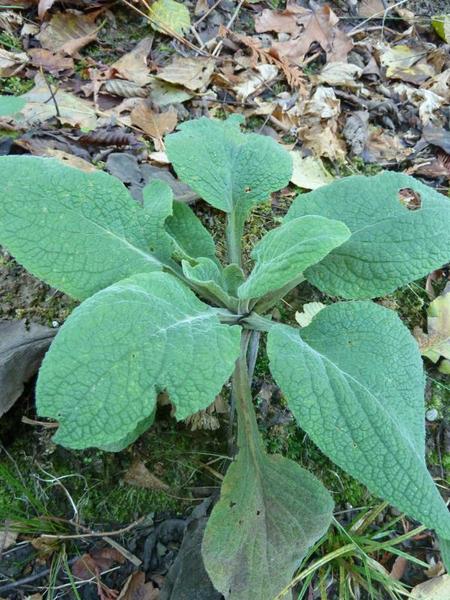
Figure 3–13. This foxglove (Digitalis purpurea) has a crown of leaves. The short distance between nodes keeps the leaves near the ground.
Kathleen Moore CC BY 2.0
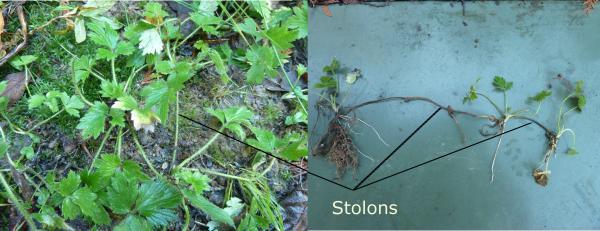
Figure 3–14. Buttercups ( Ranunculus sp.), a common weed, form long stems (stolons) between daughter plantlets making them difficult to remove.
Kathleen Moore CC BY 2.0
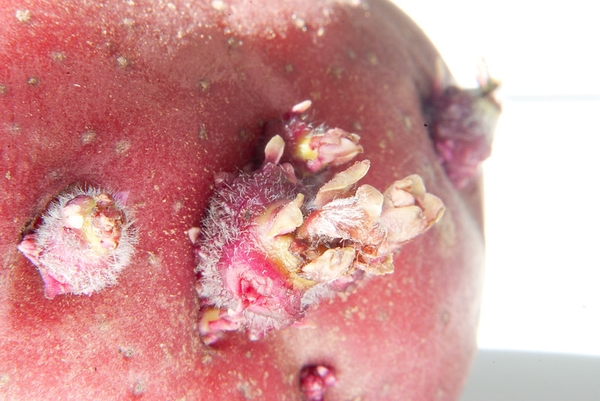
Figure 3–17. A tuber is a fleshy modified stem with nodes that produce leaves.
Steve Johnson, Flickr CC BY 2.0
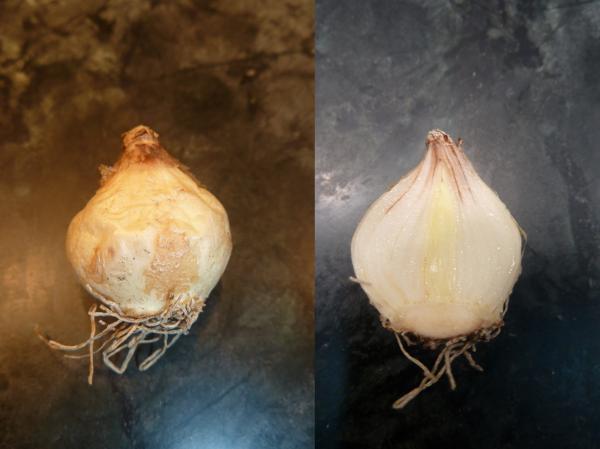
Figure 3–20. A grape hyacinth (Muscari armeniacum) bulb - whole (left) and in cross-section (right).
Kathleen Moore CC BY 2.0
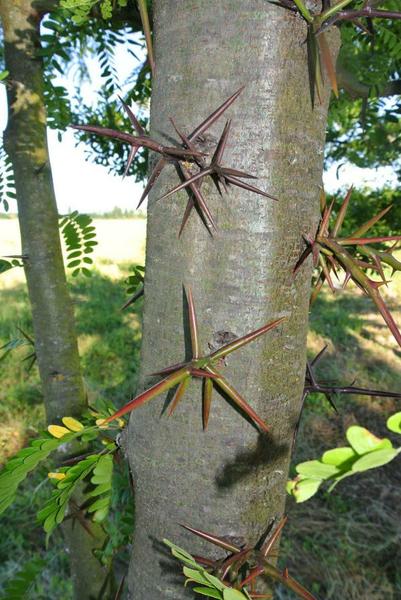
Figure 3–22. Thorns come from modified stems as seen here on this honey locust tree (Gleditsia triacanthos).
Dick Culbert, Flickr CC BY 2.0
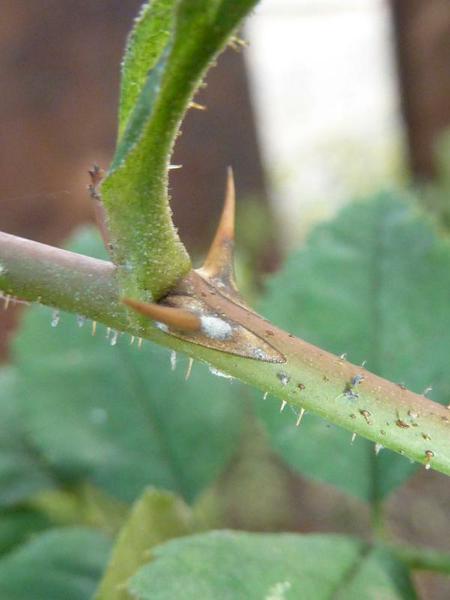
Figure 3–23. Spines are modified leaf parts. In this example, the spines are modified stipules.
Kathleen Moore CC BY 2.0
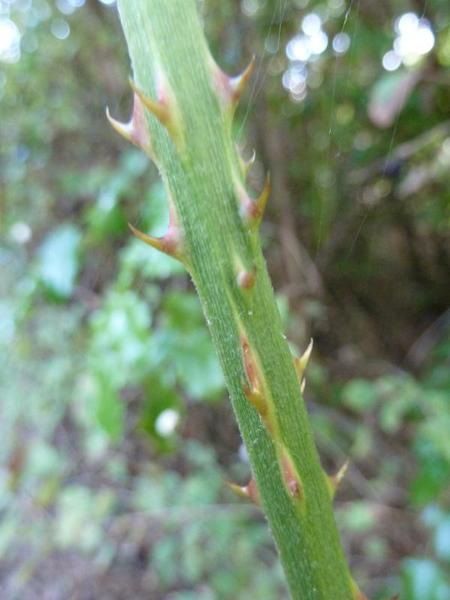
Figure 3–24. A prickle arises from an epidermal cell and can appear anywhere along the stem.
Kathleen Moore CC BY 2.0
.jpg)
Figure 3–26. A leaf bud on a stem contains many small leaves (left). Leaves and stem emerge from the bud (right).
Kathleen Moore CC BY 2.0
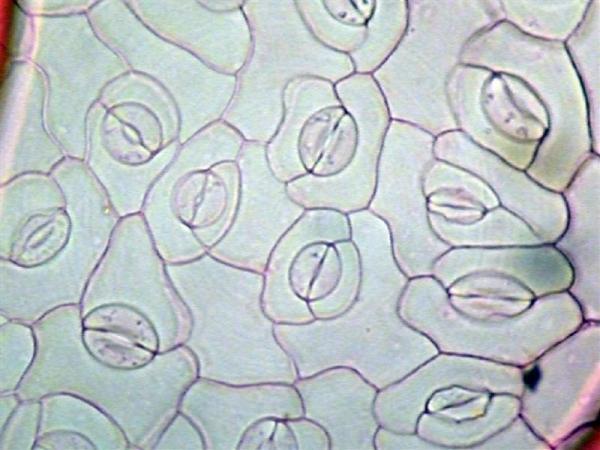
Figure 3–29. This image shows several stomatal complexes-openings on the underside of the leaf surface surrounded by two kidney-shaped guard cells. They control the exchange of gasses (such as carbon dioxide, oxygen, water vapor) by opening or closing.
Andrea Scauri, Flickr CC BY 2.0
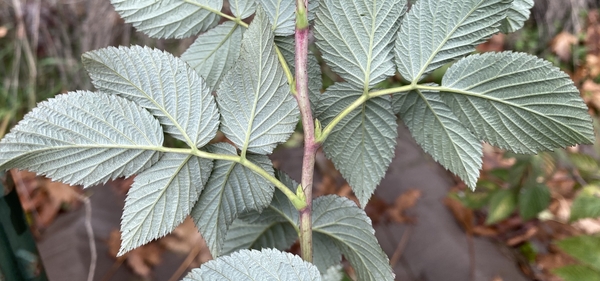
Figure 3–30. A compound leaf has several leaflets. Note the large axillary buds at the base of each leaf.
Kathleen Moore CC BY 2.0
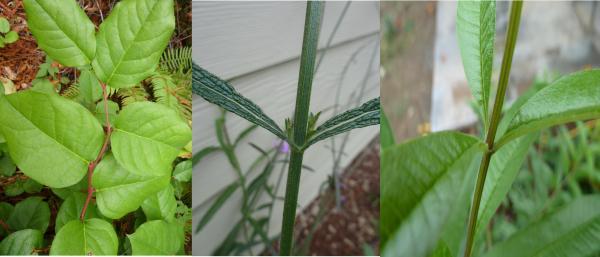
Figure 3–32. Leaves can be arranged on a stem alternately (left), opposite (middle), or in whorls (right).
Kathleen Moore CC BY 2.0
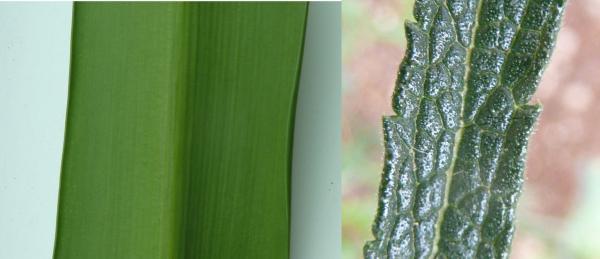
Figure 3–33. Parallel veins (left) are often seen on monocot plants, netted veins (right) are seen on dicots..
Kathleen Moore CC BY 2.0
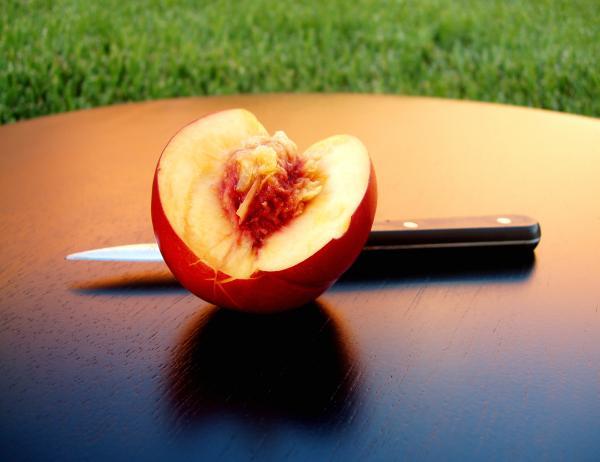
Figure 3–40. A drupe like this nectarine has a single hard seed inside the fleshy ovary.
Mary, Flickr CC BY 2.0
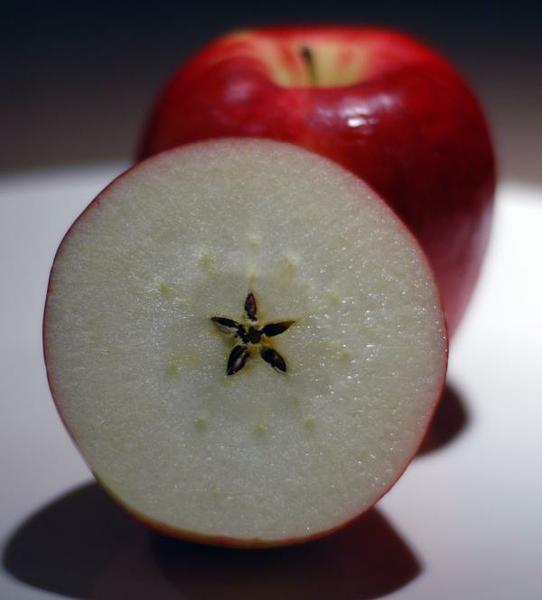
Figure 3–41. An apple is an example of a pome. In cross section the seeds form a 5-pointed star.
Denise Cross, Flickr CC BY 2.0
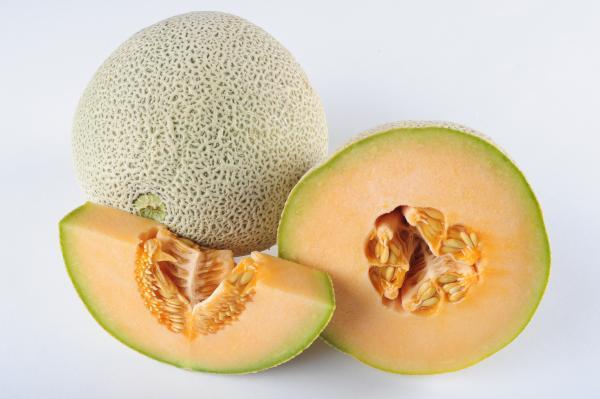
Figure 3–42. A cantaloupe is an example of a berry, a fleshy ovary with many seeds inside.
Brandon Quester, News 21, Flickr CC BY 2.0
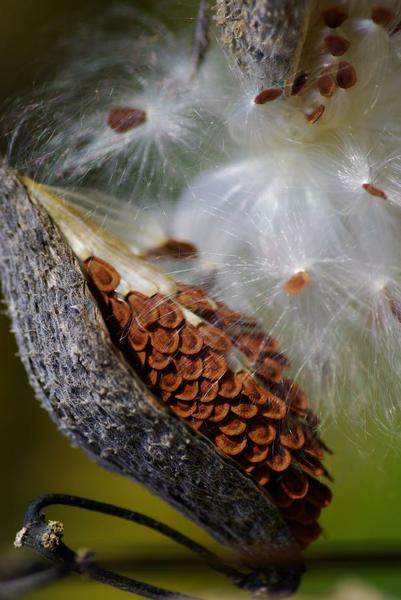
Figure 3–43. This milkweed is an example of a dehiscent or dry fruit that splits open with seeds inside.
Sarah Nystrom, U.S. Fish and Wildlife Service, Flickr CC0
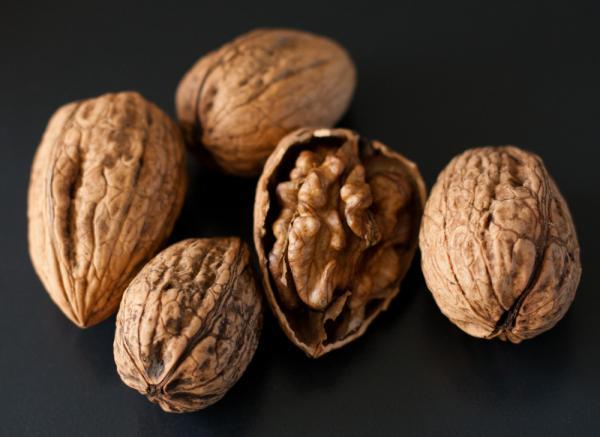
Figure 3–44. A walnut is an indehiscent fruit, dry outside and remains closed even when ripe. It is broken open either by predation or decay.
Pauline Mak, Flickr CC BY 2.0
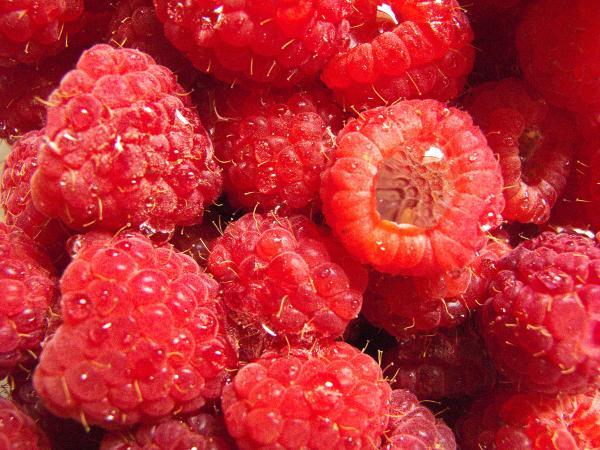
Figure 3–45. A raspberry is an aggregate fruit composed of several drupes fused together.
Ray Bodden, Flickr CC BY 2.0
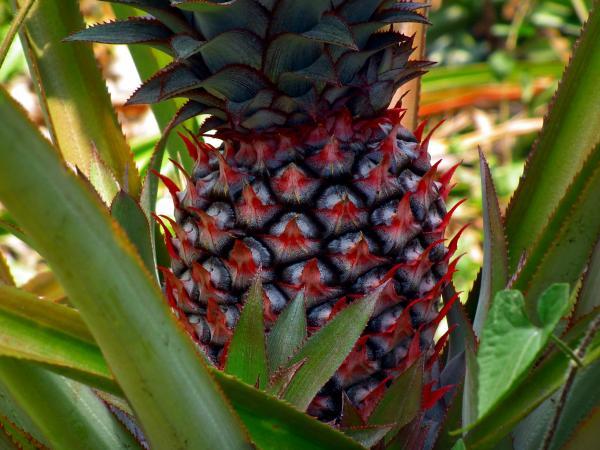
Figure 3–46. A pineapple is an example of a multiple fruit where several flowers are fused together to make one fruit.
Ramesh NG, Flickr CC BY-SA 2.0
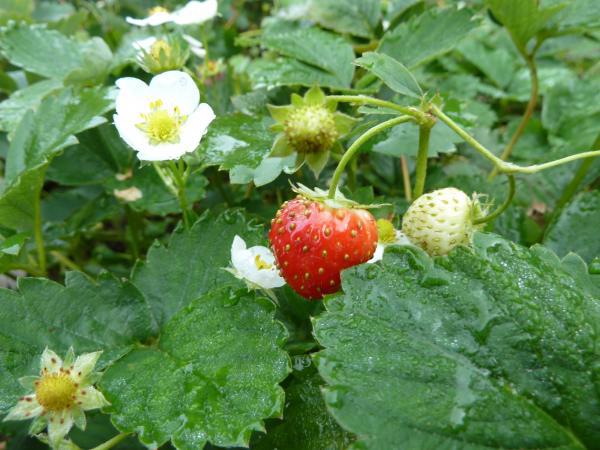
Figure 3–47. A strawberry is a ripened receptacle with seeds on the outside.
Kathleen Moore CC BY 2.0
Plant Physiology—Internal Functions and Growth
Plants perform three basic processes that drive their growth and development:
Plant growth is affected by how plants balance these three functions to survive in different environmental conditions, including various temperatures, day lengths, weather conditions, and water availability.
Photosynthesis
Unlike animals, plants can internally manufacture their own food. Through a process called photosynthesis, plants use energy from sunlight, carbon dioxide from air, and water from the soil to produce simple carbohydrates. These carbohydrates (such as glucose) are converted by different processes to complex sugars that can be transported to the stems, roots, flowers, and developing tissues for immediate use. These sugars can also be stored (as starch, the primary storage carbohydrate in plants) or used as building blocks for more complicated structures, such as oils, pigments, proteins, and cell walls.
Table 3–3. A comparison of photosynthesis and respiration.
At first glance, the equation for respiration appears to be the opposite of that used to depict photosynthesis, but respiration is not photosynthesis in reverse. It is appropriate, however, to think of photosynthesis as a carbon-building process and respiration as a process that breaks down carbon compounds to release the potential energy stored in them.
Photosynthesis | Respiration |
---|---|
uses water | uses food for plant energy |
uses carbon dioxide | produces carbon dioxide |
releases oxygen | uses oxygen |
produces food | produces water |
stores energy | releases energy |
occurs in cells containing chloroplasts | occurs in all living cells |
Photosynthesis
Photosynthesis, which literally means "to put together with light," can be described with the following chemical equation:
Carbon dioxide + Water + Light → Sugar + Oxygen + Water
The chemical notation for that equation is as follows:
6CO2 + 12H2O → light captured by chlorophyll→ C6H12O6 + 6O2 +6H2O
You can see from this equation that chlorophyll, a green pigment contained in the chloroplasts, is a necessary ingredient in photosynthesis. Although any green plant tissue can capture light energy and accomplish photosynthesis, leaves are generally the site of most food production because of their specialized structures. Their internal tissues (mesophyll) contain cells with abundant chloroplasts in an arrangement that allows for easy movement of air and water. A leaf’s stomata allow for the diffusion of carbon dioxide (CO2) into the leaf, and the CO2 is used in the manufacture of carbohydrates.
Respiration
The carbohydrates produced by photosynthesis are converted into energy for cellular work via a chemical process called respiration. It is similar to burning wood or coal to produce heat but is instead a slow and controlled release of energy. Respiration results in the formation of ATP (adenosine triphosphate), a molecule used in several metabolic reactions in plant cells to carry out cellular work. ATP is sometimes referred to as the “energy currency of life.” Respiration in cells may be shown most simply by this equation:
Sugar + Oxygen → Carbon Dioxide + Water + ATP
C6H12O6 +6O2 →6CO2 + 6H2O + ATP (energy)
Respiration occurs in all life forms and in all cells. The release of accumulated carbon dioxide and uptake of oxygen occur at the cellular level. In animals, blood carries both carbon dioxide and oxygen to and from the atmosphere by means of either lungs or gills. In plants, there is simple diffusion of carbon dioxide and oxygen into the open spaces within the leaf, and exchange occurs through the stomata. Respiration continues in flowers, fruits, and vegetables even after they are harvested. That is why refrigeration and humidity control can be used to slow respiration and prolong the shelf life of produce.
Transpiration
We have learned that plants contain vascular tissue called xylem that transports water from the tips of roots to the tips of shoots. This water forms a continuous column in the plant, which helps keep the plant upright and firm or turgid. This cell firmness is called turgor pressure. Transpiration is the process by which a plant loses water, primarily through its stomata, pulling more water up the xylem. Approximately 90% of the water taken up by a plant is lost through transpiration. The other 10%of the water is used in chemical reactions and in plant tissues.
Transpiration is necessary for:
- Transporting minerals from the soil to the plant's parts
- Cooling plant parts through evaporation
- Moving sugars and plant chemicals, such as plant hormones
- Maintaining turgor pressure (wilting is a loss of turgor)
The rate of transpiration depends on several environmental factors. An increase in light, temperature, or wind, or a combination of these factors, increases transpiration to a point. Increases in humidity, however, decrease transpiration.
Leaves of many plants begin to wilt when the rate of water loss by transpiration is greater than the rate of water absorption. As the volume of water in the plant cells is reduced, leaves and herbaceous stems become soft or flaccid. The stomata generally close during drought or high temperature stress, thus reducing further water loss. Wilting reduces transpiration losses to an extent by shrinking the affected leaf surface. The cells will expand and return to their pre-stress size and become turgid (have high turgor pressure) when the plant absorbs sufficient water.
The rate of photosynthesis decreases when stomata are closed due to reduced water availability. Not only is water less available for metabolic reactions, but carbon dioxide cannot diffuse into the leaf tissue from the atmosphere. Cell shrinkage significantly affects other essential metabolic processes as well. Therefore, temporary wilting can be harmful to the plant. Frequent wilting and slow recovery under dry soil conditions can significantly reduce plant growth. This phenomenon accounts for the reduced size of plants when a water shortage occurs. During times of drought, most crops never reach their full potential.
Environmental Factors
Plants balance their basic life functions of photosynthesis, respiration, and transpiration to grow and reproduce. Environmental factors such as light, temperature, humidity, water, carbon dioxide, and oxygen levels can influence how plants perform these life functions. Understanding how these environmental factors can affect plants enables better prediction, prevention, diagnosis, and management of plant problems.
This section will review how the following environmental factors can influence plants:
- Light
- Temperature
- Water
- Oxygen
- Carbon dioxide
Light
Light, for the purpose of photosynthesis, has three main characteristics: duration, quantity, and quality. Duration is the length of time light is present. Light quantity refers to the intensity or concentration of sunlight and varies with the season. Summer brings the most intense light, and winter the least.
Light quality refers to the colors or wavelengths of light reaching the plant surface. Sunlight can be broken up by a prism into the visible color spectrum of red, orange, yellow, green, blue, indigo, and violet (Figure 3–49). Red and blue light have the greatest effect on photosynthesis. Plants mostly reflect green light, resulting in the green color we observe and associate with them. Blue light is primarily responsible for promoting vegetative growth. Red light, when combined with blue light, promotes flowering. Plants also perceive and are affected by wavelengths of light outside of the visible color spectrum, including ultraviolet and far-red.
Fluorescent and incandescent plant lights or "grow" lights have a mixture of red and blue wavelengths that attempt to imitate sunlight as closely as possible. Fluorescent light, particularly cool-white tubes, can be used to encourage leafy growth. Such light is excellent for starting seedlings. Incandescent light is high in the red-orange wavelength ranges but generally produces too much heat.
Temperature
Temperature affects a plant’s productivity and growth by influencing flowering and fruit set, photosynthesis and respiration, water use, and dormancy. Thermoperiodism is a term used to describe the length of time that plants require exposure to certain temperatures in order to promote or inhibit different developmental events in the life cycle.
Temperature and Plant Growth
-
Photosynthesis increases as temperature increases, to a point.
-
Respiration rapidly increases as temperature increases.
-
Transpiration increases as temperature increases.
-
Flowering can be partially triggered by temperature.
-
Sugar storage increases as temperatures cool and the plant uses less energy.
-
Dormancy occurs during a period of low temperature. As temperatures warm, the plant will break dormancy and resume active growth.
- Flowering and Fruit Set—If temperatures are high and day length is long, cool-season crops such as spinach will flower prematurely (bolt) rather than produce the desired foliage. Adverse temperatures can also cause stunted growth and poor quality. For example, the bitterness in lettuce and cucumbers is caused by high temperatures. In addition, at high temperatures pollen may not be viable, preventing fruit set. On the other hand, temperatures that are too low for warm-season crops, such as tomatoes, will also prevent fruit set. Manipulating temperature, alone or in connection with day length, can control when certain flowers bloom. Chrysanthemums, for example, will flower for a longer period if daylight temperatures are 50°F. The Christmas cactus forms flowers as a result of short days and low temperatures. Daffodils can be forced to flower by putting bulbs in cold storage in October at 35 to 40°F.
- Photosynthesis and Respiration—Temperature also influences the rates of photosynthesis and respiration; those rates generally increase as the temperature rises. Very high temperatures at night, however, sometimes cause the rate of respiration to surpass that of photosynthesis. When this occurs, the plant is using food faster than it can produce food. For most plants, photosynthesis occurs at its highest rate in the temperature range of 65 to 85°F and decreases when temperatures are above or below this range. As a result, the most efficient thermoperiod (daily temperature range) for plants is usually when day temperatures are 10 to 15°F higher than night temperatures. This temperature range allows plants to photosynthesize and respire (break down food) during the day but slows down respiration at night when plants are not getting any light and hence cannot produce food.
- Water Use—Extremes in temperature can be detrimental to how plants use water. Very hot temperatures cause plants to lose water faster than they can absorb it, causing wilting and potentially stunting growth. Very low temperatures can freeze the soil, which severely restricts the movement of water into the plant. On a windy, sunny winter day, plants continue to transpire (give off water) and may become desiccated (dried out). Freezing temperatures may also result in the formation of ice crystals in and between plant cells, which can cause significant physical damage in addition to dehydration.
- Dormancy—Temperature affects plant dormancy. The buds of many plants require exposure to a certain number of days below a critical temperature (chilling hours) before the buds will resume growth in the spring. Peaches are a prime example. Most cultivars require 700 to 1,000 hours below 45°F and above 32°F before they break dormancy and begin to grow. The time period varies for different varieties. During dormancy, buds can withstand very low temperatures. But after the rest period is satisfied, buds become more susceptible to late cold or frost and can be easily damaged by these conditions. So, while very low temperatures can harm nonhardy plants, unseasonably warm winter temperatures can cause premature bud break in some plants and consequent budfreezing damage when temperatures dip back to their normal lows.
Water
Water has several important functions in photosynthesis. First, it maintains a plant's turgor, or the firmness or fullness of plant tissue. Turgor pressure in a cell can be compared to air in an inflated balloon. Water pressure, or turgor, is needed in plant cells to maintain shape and ensure cell growth (Figure 3–50). Second, water is split into hydrogen and oxygen by the solar energy that has been absorbed by chlorophyll in the plant's leaves. Oxygen is released into the atmosphere, and hydrogen is used in manufacturing carbohydrates. Third, water dissolves minerals from the soil and transports them upward from the roots and throughout the plant, where they serve as raw materials for the growth of new plant tissues. The soil moisture surrounding a plant should fluctuate. The soil should be well moistened and then allowed to dry out before irrigating again.
Oxygen
Plants use oxygen for respiration to create energy by breaking up the sugars created in photosynthesis. Oxygen is plentiful for the aboveground parts of a plant. Oxygen can be a limiting factor for a plant’s seeds, roots, and other underground parts.
Some soils contain large pore spaces that allow air and water to move freely. Well-aerated soils provide the oxygen that seeds need to germinate and roots need to grow. Some soils are dense, with poor drainage, leaving little to no pore space for oxygen. Soils that are oversaturated with water or compacted can prevent access to oxygen and cause root death (Figure 3–51). Read more about the importance of soil structure in chapter1, “Soils and Plant Nutrients.”
Most roots occur in the upper foot or two of soil because that is where the most oxygen is located. The deeper the soil is, the less oxygen there is. Plants grown in saturated soils often have roots growing on the soil surface.
Carbon Dioxide
Carbon dioxide is a key component in photosynthesis. Carbon dioxide is plentiful, and its quantity in the air is rarely a limiting factor for plant growth. Most plants take in carbon dioxide through open stomata during the day when sunlight is available and photosynthesis is actively occurring. Carbon dioxide can be a limiting factor if the plant does not open its stomata during the day. Photosynthesis slows and can stop completely when stomata close. Stomata may close during the day to prevent water loss if the plant is drought stressed.
Soil
Another environmental factor is the soil. Soil structure, texture, and nutrient availability each have environmental impacts on plants. For more information about soils, see chapter 1, “Soils and Plant Nutrients.”
Exceptions to the rule:
Some desert-adapted plants, such as cacti and succulents, perform a different type of photosynthesis known as CAM or Crassulacean acid metabolism (Figure 3–52). CAM allows plants to keep their stomata closed during the hot part of the day to prevent water loss. These plants open their stomata at night and save the collected carbon dioxide for the next day when sunlight is available.
Plant Growth Chemicals
Hormones are chemicals that allow a plant to “communicate” with itself in response to the environment. Hormones exist in small concentrations in a plant, but they can have a big impact on its growth, health, and reproduction. Any cell in a plant can produce hormones, but they are typically produced in a plant’s meristem. Hormones can travel through cells by osmosis, but they often travel down the plant from leaves to roots via phloem cells or up the plants from roots to leaves via xylem cells. Understanding the basics about plant hormones helps us understand why plants react to stimuli and how a change in hormone levels can encourage desired or undesired responses in a plant.
Plant growth is regulated by several classes of hormones: auxins, cytokinins, gibberellins, ethylene, and abscisic acid.
- Auxins are produced in the stem tips and promote apical dominance by suppressing the growth of lateral buds. Removing the terminal bud (pinching or pruning back a plant) eliminates the source of auxin, and thus allows lateral buds to grow. In addition, auxins can induce adventitious root formation on cuttings. Auxins differentially support cell division and cell elongation, resulting in directed growth in response to environmental factors. This is called tropism. The directional growth response to gravity is called geotropism, and the growth response to light is called phototropism (Figure 3–53). A seed will send its roots down into the soil and its shoots up toward the soil’s surface due to gravitropism. A plant will grow away from darkness by elongating cells on the side of the plant in the dark. People often say that plants grow “toward the light,” but the elongation of cells is actually a growing away from darkness. Auxins also inhibit the dropping of leaves, flowers, and fruits (a process called abscission), and stimulate ethylene production.
- Cytokinins promote cell division. They enhance shoot growth and counteract the effect of auxins in apical dominance. Cytokinin levels increase in axillary buds when apical dominance is removed. Cytokinins also help tissues delay senescence, the aging and maturity of tissues that ultimately results in tissue death. In short, auxins promote vertical (apical) growth; cytokinins promote axillary (lateral) growth.
- Gibberellins increase cell division, promote internode elongation, and regulate the production of seed enzymes. These enzymes are important during germination because they break down storage carbohydrates and proteins for use by the embryo. Gibberellins also stimulate flowering and can increase leaf size. Gibberellins can be used to force camellias to bloom out of season and to regulate the production of seedless grapes. A single treatment will more than double the size of Thompson seedless grapes and create a looser cluster of fruits. Gibberellins can be used to coax a plant out of dormancy and to green up a lawn two to three weeks earlier than normally.
- Ethylene is the only hormone that is a gas. It speeds aging of tissues and enhances fruit ripening (Figure 3–54). Fruits such as apples and bananas produce large quantities of ethylene. Enclosing a fruit that is a mature size but still green, such as a tomato, in a bag with some apples will speed the ripening of the tomato. Ethylene is produced in almost every condition in which plant tissues are stressed, physically and chemically. It is also the primary abscission hormone in plants and is responsible for generating an abscission layer at the base of leaves in deciduous species when they drop their leaves. Ethylene is generally associated with senescence of plant tissues and organs.
- Abscisic acid (ABA) is considered a “stress hormone.” It is a signaling molecule that induces stomatal closure during a drought or high-temperature stress conditions. ABA is involved in dormancy of seeds and buds and promotes abscission (loss of leaves, buds, or fruit). It protects the plant by inhibiting growth during times of stress.
Gardeners can influence plant hormones through plant modification or through the use of plant growth regulators. Any pruning will modify a plant’s hormone balance and cause a change in growth pattern. If you remove the leggy stems of a mint plant, you will be cutting back auxin levels from the apical meristem dominance. Cytokinin levels will increase, stimulating “bushy” lateral meristem growth and creating a fuller, more attractive plant. On the contrary, if you pinch back the side branches of your tomato plants, you will reduce the cytokinin levels and encourage apical dominance from auxins. This apical dominance will reduce the number of tomato fruits but will increase fruit size.
A chemical that mimics a hormone is known as a plant growth regulator. Plant growth regulators are applied to plants for a specific purpose to achieve a desired result. Many production nurseries use plant growth regulators in their greenhouses, and farmers use them in their agricultural fields to increase plant quality and crop yield. Garden centers sell the following growth regulators:
- Auxin rooting hormone can be used in propagation to stimulate the growth of roots from stem cuttings. These products are sold as indoleacetic acid (IAA), indolebuytric acid (IBA), or naphthalene acetic acid (NAA). The product for woody plant stem cuttings is 1-napthaleneacetimide (NAD).
- Some auxin products can kill broadleaf plants without harming monocots (grass).
- Gibberellic acid is used as a cut-flower preservative and to increase shoot growth, increase fruit size, and hasten maturity.
- Ethylene products enhance fruit color and hasten ripening.
Notching an Apple Tree to Encourage Lateral Growth
Sometimes young fruit trees need encouragement to produce a quality canopy. If you have a fruit tree with a central leader that could use some more side branches, you can encourage lateral growth by notching. Notching will encourage the canopy to fill in without major branch loss due to heading cuts. Two to three weeks before bloom, find the buds along the leader. Use a small hacksaw to make shallow cuts a third of the way around the branch just above the nodes where branches are desired. Be sure to leave some nodes for later, in case a limb is lost. Cut only through the phloem, not the structural part of the branch. This notching will disturb the flow of auxin hormone from the central leader and cause the axillary meristems to sprout and grow.
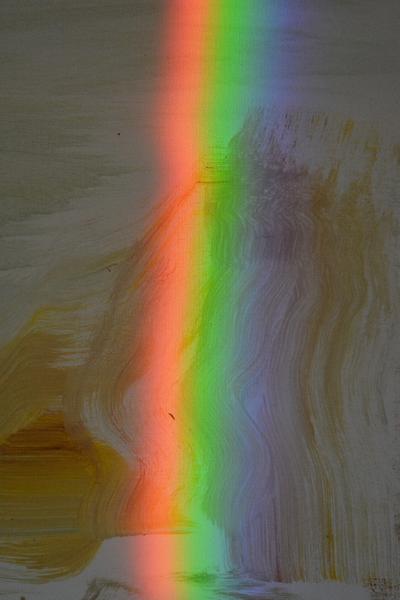
Figure 3–49. The visible spectrum of sunlight: red, orange, yellow, green, blue, indigo, and violet.
Aiko Van Hulsen, Flickr CC BY 2.0
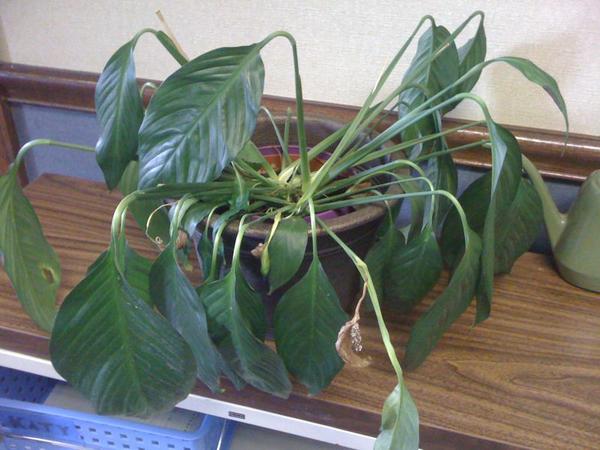
Figure 3–50. Turgor pressure in cells is kept up by adequate water. This peace lily (Spathiphyllum spp.) is wilting (loss of turgor pressure) from lack of water.
Cynthia Closkey, Flickr CC BY 2.0
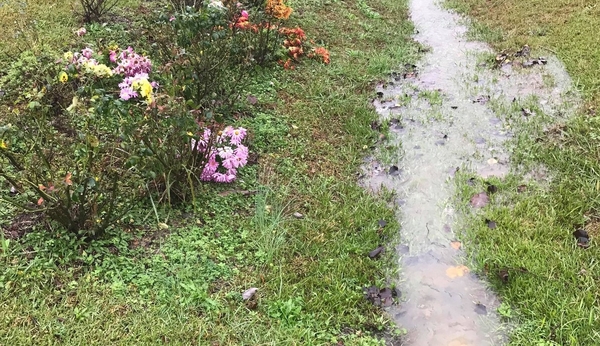
Figure 3–51. These shrubs are planted in dense soil, causing water to pool after a heavy rain. Oversaturation is detrimental to the shrubs' roots by reducing pore space and oxygen.
Emily LeaAnn, Flickr CC0
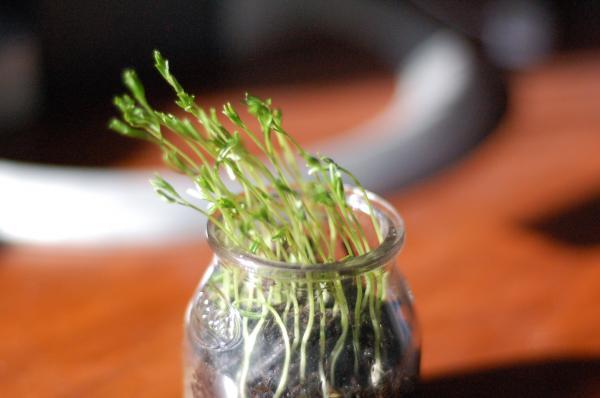
Figure 3–53. Phototropism: auxins accumulate on the dark side of a stem, elongating those cells so that the shoot tissues grow towards a light source.
Russell Neches, Flickr CC BY 2.0
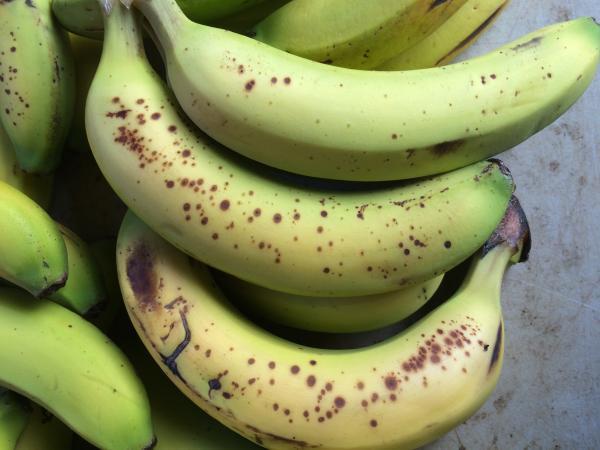
Figure 3–54. These spots indicate stage 7 of ripening (overripe), which can derive from too much exposure to ethylene gas at a ripening facility.
Scot Nelson, Flickr CC0
Frequently Asked Questions
1. A tree branch grows over my sidewalk, and people keep hitting their head on it. Should I wait for the tree to grow, or should I cut off the branch?
Plants continue to grow throughout their life at places on the plant known as meristems, which are located as follows:
- The tips of branches
- The tips of roots
- In bud tissues at nodes
- The vascular cambium tissue in the trunk (makes the trunk get thicker each year)
If the branch is too low to the ground, none of these meristematic areas of growth are going to raise this branch any higher off the ground. If the branch is a hazard, remove it. Follow the branch back to the union. Remove the branch at its point of attachment so it can compartmentalize the wound. Use sharp, sterilized pruning tools. If it is a large branch, be sure to make a three-point cut. For more information about pruning, see chapter 11, “Woody Ornamentals.”
2. My plants didn’t flower this year. Why?
A plant may not flower for many reasons:
- The plant has experienced some stress, either lack of water, nutrients, or light.
- It could be immature. Some plants take several years before they reach sexual maturity.
- Excess nitrogen fertilizer can cause leaf growth instead of flower growth.
- Flower buds can be very sensitive to frost. Cold weather during any time of bud development can hinder flowering.
- Incorrect pruning at the wrong time can remove flower buds, especially if a plant flowers on last year’s wood. See chapter 11, “Woody Ornamentals”, for information on the correct time to prune.
3. My muscadine grapevine does not have any fruit, or my muscadine has small fruit that did not ripen. There are many reasons for poor yield in grapevines:
- Does it get enough sun? Fruit set and production will be reduced if the vines are shaded for more than several hours a day.
- Have you been giving it a high nitrogen fertilizer, which could cause leaf growth at the expense of fruit development?
Submit a soil sample to obtain specific fertilizer recommendations. Simply adding a balanced fertilizer, without knowing what your soil needs, can lead to high phosphorus in the soil. Boron deficiency may also result in poor fruit set, especially on sandy soils with high pH. Submit a foliar sample to determine boron status.
Further Reading
Capon, Brian. Botany for Gardeners. 3rd ed. Portland, Oregon: Timber Press, Inc., 2010. Print.
Coombes, Allen J. The A to Z of Plant Names: A Quick Reference Guide to 4,000 Garden Plants. Portland, Oregon: Timber Press, Inc., 2012. Print.
Hodge, Geoff. Practical Botany for Gardeners: Over 3,000 Botanical Terms Explained and Explored. Chicago: The University of Chicago Press, 2013. Print.
Johnson, Arthur T., and H. A. Smith. Plant Names Simplified. 2nd ed. Ipswich, England: Old Pond Publishing Ltd., 2008. Print.
Radford, Albert E., Harry E. Ahles, and C. Ritchie Bell. Manual of the Vascular Flora of the Carolinas. Chapel Hill, North Carolina: The University of North Carolina Press, 1968. Print.
Stearn, William T. Botanical Latin. 4th ed. 1992. Portland, Oregon: Timber Press, Inc., 2004. Print.
Staff of the Liberty Hyde Bailey Hortorium, Cornell University. Hortus Third: A Concise Dictionary of Plants Cultivated in the United States and Canada. New York: McMillan Publishers, 1976. Print.
For More Information
NC State Resources
More NC State Resources
Other Resources
- LatinTutorial
- Plant Morphology – Leaves and Flowers, by American Museum of Natural History
- PLANTS Interactive ID Keys, USDA
- “Science and conservation”, Kew Royal Botanic Gardens
Contributors
Contributions by Faculty:
Chad Jordan, Professor, Undergraduate Programs Coordinator, NC State Department of Plant Biology
Contributions by Extension Agents: Thomas Glasgow, Amy-Lynn Albertson, Charlotte Glen, Ben Grandon, and Carl Matyac
Contributions by Extension Master Gardener Volunteers: Patty Brown, Karen Damari, Margaret Genkins, Renee Lampila, Edna Burger, Connie Schultz, Barbara Goodman, Caro Dosé, and Jackie Weedon
Content Editors:
Lucy Bradley, Associate Professor and Extension Specialist, Urban Horticulture, NC State University; Director, NC State Extension Master Gardener program
Kathleen Moore, Urban Horticulturalist
Copy Editor:
Barbara Scott
Based in part on text from the 1998 Extension Master Gardener manual prepared by:
- Erv Evans, Former Extension Associate, Department of Horticultural Science
- Paul Fantz, Professor Emeritus, Department of Horticultural Science
- James Hardin, Professor Emeritus, Department of Botany
Adapted in part from the Virginia Gardener Handbook.
How to cite this chapter:
Moore, K.A., and. L.K. Bradley (eds). 2022. North Carolina Extension Gardener Handbook, 2nd ed. NC State Extension, Raleigh, NC. <http://content.ces.ncsu.edu/3-botany>
Publication date: Feb. 1, 2022
AG-831
Other Publications in North Carolina Extension Gardener Handbook
- 1. Soils & Plant Nutrients
- 2. Composting
- 3. Botany
- 4. Insects
- 5. Diseases and Disorders
- 6. Weeds
- 7. Diagnostics
- 8. Integrated Pest Management (IPM)
- 9. Lawns
- 10. Herbaceous Ornamentals
- 11. Woody Ornamentals
- 12. Native Plants
- 13. Propagation
- 14. Small Fruits
- 15. Tree Fruit and Nuts
- 16. Vegetable Gardening
- 17. Organic Gardening
- 18. Plants Grown in Containers
- 19. Landscape Design
- 20. Wildlife
- 21. Youth, Community, and Therapeutic Gardening
- Appendix A. Garden Journaling
- Appendix B. Pesticides and Pesticide Safety
- Appendix C. Diagnostic Tables
- Appendix D. Garden Tools
- Appendix E. Season Extenders and Greenhouses
- Appendix F. History of Landscape Design
- Appendix G. Permaculture Design
- Appendix H. Community Gardening Resources
- Appendix I. More NC State Resources
- Glossary
N.C. Cooperative Extension prohibits discrimination and harassment regardless of age, color, disability, family and marital status, gender identity, national origin, political beliefs, race, religion, sex (including pregnancy), sexual orientation and veteran status.